Here is a video if I can upload it to say I have now managed a decade as a science and disease sceptic and with an unconventional view of animal intelligence.
L Orrock: medical science is science fiction
Tuesday, March 14, 2023
Thursday, October 29, 2020
New essay arguing that microscopes don't work
I'll post it when I can. I can't find a way of uploading from my chromebook presently. I see I posted a photo or video of worms emerging from rotting oranges. Since my post last year I have also realised bathroom slugs (out at night only) are emerging from the damp/dirt - not all of it necessarily my neglect - between the tiles.
Prisoner of whore - I am having trouble posting updates.
Friday, November 8, 2019
Snell's Law and de Broglie's equation - draft points
Is refraction intended to be a valid explanation of apparent magnification in the traditional and electron microscopes? A consideration of Snell’s Law and de Broglie’s equation.
1.In traditional optics, how would refraction, or the bending of a light ray as it enters a different medium, account for an enlarged image? What is apparently being stretched in some way is the object, in the sense that although it is called an image it is a real one because we are viewing it directly, not a virtual image projected onto a screen or, for instance, captured within the lens. But is this conceivable, or does it ‘stretch’ credulity?
2. Is the term 'normal' supposed to lead us to think about the fact that even if light does bend and this has an effect on the size of the object/image we view, it would also bend back to the angle at which it entered the lens, presumably, as it left it? (And the use of the term ‘ordinary glass’ sometimes used with the unit of refractive index might be to imply that at least one of the lenses in the cylinder, the objective lens, is in fact just that, plane glass rather than a convex lens.)
3. The calculation used to find out the two different angles, before and after it changes medium, is Snell’s Law: sin(e) of the incident angle x refractive index = sin(e) of the refracted angle x refractive index. Refractive index is related to the speed at which light travels in a particular medium but inversely, so that it is higher for a lens than in air. If the angle of transmission, or refraction, bends towards the 'normal' - ie, towards the perpendicular - in the denser glass of the lens with traditional optics, how does this result in an enlarged image? By analogy, would a light projector that shone inwards rather than outwards as it left the projector result in a larger or smaller image on the screen ahead of it? If the light source is underneath the lens, as it is, for instance with the Apex Learner, then in what sense is the object or its image enlarged beyond the microscope? Would we not be seeing exactly the same image without the aid of the microscope?
4. However, with electron optics - which replaces light with electron beams and ordinary lenses with magnetic lenses - the variable in Snell’s Law is not refractive index but velocity itself. If the direction of the change of angle is the opposite that we would expect with traditional light refraction, should we really still consider what is taking place to be refraction? If we replaced a red light with a green one we would not expect the angle to increase rather than decrease. If the property of electron beams is such that a change of medium would produce the opposite effect to that of light this is not, as far as I have read so far, asserted or explained.
5. Would a difference in refractive index of only about 1.5 in traditional optics make enough difference in the angle to account for significant magnification? In other words, assuming the difference in angle were that of 60° and 90°, which would be the case if light approached the lens at 90°, would we then much more in the way of enlargement than a ratio of 3:2, assuming an effect of enlargement rather than reduction. (See point .. below.) If, however, we imagine a larger angle as it enters the lens, which is said to be the case with electron optics, then were the beam to reach the glass at 60° and it refracted to 90° , then would the result be diffraction or an image infinitely large? And what if the difference in refractive index were not 1.5 times but the 1000 times said to be possible in electron optics?
6. I have so far set to one side the fact that the variable was sine of the angle rather than the angle itself. However, although a correct calculation leads to variations the numbers are not extremely significant. For example, an angle of 30 in air will result in an angle of approximately 20 in the lens, which is almost the same as we would expect if we were doing the calculation with angles rather than sine.
0.5 x 1 = 0.33 x 1.5.
If we replace the 30 beam of light with one approaching the lens at 90, however, the equation is the following:
1 x 1 = 0.67 x 1.5,
Whereby the refracted angle, however, is approximately [45], not 60. While the change in the angle is proportional to the increase in sine, the difference is greater than if we were using the angle alone. However, since the difference is greatest at 90 [check] and is of a ratio of only 2:1 rather than 3:2, the difference in terms of enlargement is not significant if one considers the stated maximum enlargement in traditional optics is 800x or more. [1 more]
7. Of greater importance, why would sine be introduced other than to put off the layperson or to demonstrate that the equation is nonsense? Trigonometry is apparently necessary to calculate sides of a triangle with respect to an angle but not the ratio of two angles where a non mathematical variable is introduced, refractive index. In most cases, also, neither will it be a case of contiguous [?] triangles since the light will rarely reach the lens at a point, but this is beside the point when the non-mathematical or applied-mathematical variable of refractive index is the second of the two variables in the equation.
8. While we might expect Snell’s Law to be the same in traditional and electron beams, would it, however, even be applicable in electron optics given that we are talking about electron beams rather than light. Refractive indices are derived from the variations in speed of light in different media. Could we necessarily assume the variations to be the same with electron beams? On the other hand, neither can the replacement of refractive index with velocity in the equation, an entirely different matter, be deduced from the replacement of light with electron beams. With electron optics there is an independent variable, acceleration, on the basis that electron beams can apparently be accelerated or decelerated, whereas light beams, whatever their source, remain at a constant speed in a given medium. However, variations in speed do not imply that the angle should decrease at a lower speed - because of a higher refractive index - rather than increase.
9. With electron optics there is an independent variable, acceleration, on the basis that electron beams can apparently be accelerated or decelerated, whereas light beams, whatever their source, remain at a constant speed in a given medium.. The electron beam is accelerated - although not beyond the speed of light - or decelerated, apparently, to produce variations in refractive indices of up to now 1,000 times. However, because the change in velocity is said to be 'continuous' rather than abrupt, again can this be considered refraction at all?
10. Again with electron optics, most of the light is described as ‘scattering incoherently’ rather than bending at a precise angle as it enters the lens. Again, is this what refraction predicts?
11. Sin(e), used to calculate the incident and refracted angle in Snell’s Law, is calculated by dividing the length of the opposite side by that of the length of the hypotenuse ('sense of humour'?). Sin(e) increases from 0 - 1 as the angle increases. Why sin(e) is included when we are not concerned with sides and there is no triangle, I am not sure, although it will not affect the difference in the angles one way or another, since the numbers are still the derivative of angles.
12. If refraction is said to be the mechanism of enlargement - rather than convexity and concavity, which however feature in diagrams of what happens in a lens - then why is there not magnification when we look through any plane glass, such as a window?
13. Why is refraction considered the mechanism of magnification, in any case, when with a magnifying glass convexity and concavity might plausibly account for magnification and this is suggested by diagrams explaining magnification? However, even if refraction was not thought to be a necessary factor, you would still need to take it into account if refraction is something that happens to light anyway; and yet in diagrams of what happens to light as it enters lenses, a light ray travels through the centre point of the lens without bending at all. However, very large differences in refractive indices are said to be the principal mechanism of magnification with electron optics and the fact remains that it is said to be the mechanism in traditional optics, so that invalidating refraction will also invalidate magnification.
14. Waves are important in optics because they reduce clarity (the ability to distinguish an object) and resolution (the ability to distinguish between objects): light travels in waves and these produce diffraction, or blurring, rather than the precise directions we are said to need when refraction produces magnification. I have so far got stuck on the equation for wavelength, not yet being able to understand the difference between the variables, h and p. (Texts I have consulted can have a habit of doing that, explaining - I assume deliberately and obviously - what one of the variables is but not the other, which in the case of this journal article you go back a few pages for and then it seems to be the same thing.) According to an entry in the 1945 Journal of Physics, wavelength is equal to h over p, momentum, where both h and p appear to be momentum, or velocity x mass).
“It is well known that a theoretical limit exists for the resolving power of an optical instrument. The smallest object that may be perceived through a microscope is limited, owing to diffraction effects, by the wavelength of the light employed. Thus while light may be considered as traveling in straight lines in many cases, the wavelike properties of light must be considered in attempting to detect very small objects. The possibility that particles should also exhibit wavelike properties was first suggested by de Broglie (see paper I). He proposed to associate with a particle of momentum p a wavelength
ƛ = h/p.”
This might explain the use of the term ‘standard wavelength’ elsewhere: ie, that according to this calculation, wavelength would always be 1. The equation was apparently proposed by de Broglie. Although the journal author appears to be deliberately introducing confusion between light and electron beam waves and that of particles that might instead be referring to the objects being viewed, he then tells us that the same equation was used by Davisson and Germer with respect to electron waves. The author also makes a link between de Broglie’s equation and Dirac’s,used to calculate the different numbers of electrons travelling at different speeds within an electron beam although the ‘thus here also’ where de Broglie’s follows Dirac’s might be taken to be ironic, at least in the ease with which it might be applied.
Waves are important in optics because they reduce clarity. However, in any case, why would the shorter wavelengths said to be associated with electron beams rather than light produce less, rather than more, diffraction? An analogy, although it might be mixing metaphors inappropriately, would be that it is easier to see one's reflection in water where there are fewer ripples than in one where there are more.
15. An entry in the American Journal of Physics mentioned 'intervening media', which - setting aside any other meaning of media intervention - led me initially to think, instead of the light or electron beam in fact traveling straight from the air to the glass of the lens or to the magnetic lens do the bits of metal and plane glass as well as air or vacuum in between the air and lens need to be taken into account when calculating refraction? However, ‘on reflection’, the reference to intervening media is more likely to be ironic in that if, for instance, one takes the eyepiece off the Apex Learner microscope one sees nothing until what looks like a bright light at the end of the objective lens tube. The 'condenser' referred to in textbooks is not apparent.
16. This quotation from a 1958 American Journal of Physics article is one of many hints that what is going on is reflection rather than refraction:
"On viewing a bright point of light through the double mirrors, multiple images of the source are seen by the eye. The angle between successive images is twice the angle between the mirrors, irrespective of the angle of incidence."
In fact, this might be suggesting that the larger image is the result only of a larger eyepiece lens compared to the size of both the objective lens tube and the circle that the object is placed on - with variation being accounted for by whether or not the screen is filled as well as by external interference and manipulation of the image.
17. Refractive index is derived from the velocity of light in the media, which is derived from what? It might be thought the other way round if one uses a protractor to measure the incident and refracted angles - an exciting new development in the measurement of Snell’s Law according to an entry in the 1958 edition of the American Journal of Physics.
Bibliography
Although it might be thought I have relied too heavily on out-of-date texts, they seem to make more obvious hints than later works, including A level textbooks, that I have consulted.
American Journal of Physics, 1945 and 1958 editions.
Hecht, Eugene. Outline College Physics, 2012.
el Kareh and el Kareh, Electron Optics, 1970.
Wikipedia entries on: refraction, the electron microscope, magnetic lenses, Dirac’s Law, momentum.
Monday, June 10, 2019
Attached is an ad about microscopes I put in a local paper in April 2019.
In fact, I can't find them, although probably other people can. I have an Acer Chromebook that hides documents and offers me the choice of finding ones I own and ones owned by everybody. It's the most awful thing to use except for browsing the internet. The job centre sent me on the European Computer Driving Licence levels 1 and 2, which were actually quite difficult to pass, but they are of no use whatsoever with the Acer chromebook. I am extremely heavily gassed when I turn on the desktop computer, although it stopped working before I went on holiday anyway.
Sometimes i wonder if the inventor of AIDS is waiting to take the credit for uninventing it.
In fact, I can't find them, although probably other people can. I have an Acer Chromebook that hides documents and offers me the choice of finding ones I own and ones owned by everybody. It's the most awful thing to use except for browsing the internet. The job centre sent me on the European Computer Driving Licence levels 1 and 2, which were actually quite difficult to pass, but they are of no use whatsoever with the Acer chromebook. I am extremely heavily gassed when I turn on the desktop computer, although it stopped working before I went on holiday anyway.
Sometimes i wonder if the inventor of AIDS is waiting to take the credit for uninventing it.
I haven't done many more observations recently although was interested when looking at a sample of blood under the Apex Learner that the image was entirely monochrome at whichever magnification. Unfortunately the slide then disappeared from the hotel room I was staying in and I haven't repeated the experiment since, although since by coincidence I see there is a bit of blood where I dropped something earlier on my foot I might try doing so later today.
I've just realised that although they say they can accelerate electron beams that then decelerate within the magnet lens in the electron microscope, the speed of ordinary light in air, rather than in a vacuum, is almost that of the speed of light in a vacuum - ie, "the speed of light "- which according to Einstein nothing could travel faster than. So they have come up with accelerators to accelerate electron beams so that they will travel as fast as light already does - if light actually does have speed - within the traditional microscope.
I have written to essays since the last post that I'll put up later. Both incorporate at least one new point, which is that Snell's Law changed with the introduction of the electron microscope. Snell's Law is used to calculate the change in the angle as light enters a different medium because of refraction. It is: angle of incidence x sin(e) x refractive index a. = angle of refraction or transmission x sin(e) x refractive index b. So that because you have a larger refractive index with a denser material you have a smaller angle when light enters eg a lens from air. It then returns to the prior angle, of course, when exiting the lens. However, with electron optics you have a new Snell's Law where the variable is not refractive index but velocity, which is inversely proportional to refractive index since the refractive index of any material is equal to the speed, or velocity, of light in air over the speed/velocity in the material. This point is laboured in the book I have been looking at so as to make it seem they are trying to make you think about it. However, what I hadn't thought of, despite references, for example, to 'slow electrons' is that nothing is supposed to travel faster than the speed of light.
I have written to essays since the last post that I'll put up later. Both incorporate at least one new point, which is that Snell's Law changed with the introduction of the electron microscope. Snell's Law is used to calculate the change in the angle as light enters a different medium because of refraction. It is: angle of incidence x sin(e) x refractive index a. = angle of refraction or transmission x sin(e) x refractive index b. So that because you have a larger refractive index with a denser material you have a smaller angle when light enters eg a lens from air. It then returns to the prior angle, of course, when exiting the lens. However, with electron optics you have a new Snell's Law where the variable is not refractive index but velocity, which is inversely proportional to refractive index since the refractive index of any material is equal to the speed, or velocity, of light in air over the speed/velocity in the material. This point is laboured in the book I have been looking at so as to make it seem they are trying to make you think about it. However, what I hadn't thought of, despite references, for example, to 'slow electrons' is that nothing is supposed to travel faster than the speed of light.
Thursday, September 6, 2018
Part of revised introduction
Here is the beginning of the revised introduction. I cannot with my Acer chromebook scroll down to find out if I can attach a file. Nor can I apparently scroll down very far on the document itself to paste and copy from it. More and more of my functions on the chromebook seem to be taken over by a hacker:
` Does the microscope achieve high enough magnification to view the ‘cell’?
“So the physicists have produced a microscope known as the Electron Microscope, which does not use light rays at all but operates with cathode rays. Instead of a beam of light it employs a stream of electrons, and electromagnets instead of lenses.” Kenneth M. Smith, Beyond the Microscope, 1945.
“[I]n 1904, Arthur Balfour announced on the part of British science that the human race without exception had lived and died in a world of illusion until the last year of the century.” The Massachusetts Historical Society, The Education of Henry Adams, An Autobiography, September 1918.
Introduction
Diagnosed diseases and the fear of them shorten and restrict lives. In 2012, having read about three unrelated cancers, I guessed that diagnosed diseases were fictitious and shortly afterwards that they were, to invert Clausewitz’s phrase, a continuation of war by other means. After completing essays on cancer and HIV in 2013, I attempted to prove that microscopes would not be able to diagnose diseases, and this is the main subject of the present essay. Because my background was not in science, I initially preferred making inferences to carrying out the observations and for this reason, and because I have not yet managed to take a microscope apart, the explanations may lack authority. I was also sure that because I could not obtain a clear image of what was beyond the microscope with the Prinz 2801, I would not be able to with any microscope and I also misunderstood the magnification claimed for the Sunagor MagnaScope. However, I think the initial observations I made with the Prinz and Sunagor MagnaScope and with magnifying glasses allowed me to draw accurate conclusions that were true, for example, that the lens is reflecting inwards rather than refracting outwards when it enlarges the object, as, for example, we can observe reflection taking place, and because standard accounts of the principle of refraction and of what happens in the lens do not appear even to be trying to describe the process coherently.
My understanding improved after I bought two further microscopes, the Apex Learner and Celestron 600, gained experience in carrying out observations and began to look at standard texts. However, the essay is still in draft form. Therefore, although certain in my conclusion I am not yet confident about what is taking place in the lens nor, for instance, of the role of the eye itself, nor of the clarity theoretically possible at high magnifications.
Also, it may be thought that much of what I have written is beside the point because electron microscopes, which have been used to diagnose disease since the 1930s, work very differently. This is especially true if I am correct in assuming the laws of refraction applicable to the microscope are the same as those for magnifying lenses, although there seems to be some evasiveness in the literature about this. In electron microscopes, the light is replaced by negatively charged electrons, and the variable is not the refractive index but the velocity of the electron. However, this seems more or less to amount to the same thing: the angle of incidence and refraction in the two materials is proportional to the square root of their ‘potential’, or velocity within the material, which in turn depends upon the two refractive indexes, the ‘square root’, I think, adding nothing to the explanation since it does not change the ratio. Neither does the explanation in this 1970 publication inspire confidence:
“[T]he quantity in electron optics which corresponds to the index of refraction in light optics is the electron velocity. But, as is well known, the electron velocity is proportional to the square root of the potential (for slow electrons, of course)”.
So far, I have also understood that the change in the angle is ‘continuous’ rather than ‘abrupt’, which may be consistent with a lower rather than higher variation, and, easier to picture but more difficult from the point of view of magnification, that a ‘substantial portion’ of an electron beam travelling through matter will be ‘scattered incoherently’. Also, the fact that the electron microscope lenses are only convergentsupports the idea that we are not seeing an object beyond the microscope and if that sounds irrelevant or incoherent because we are viewing an image on a computer screen then I think we still at least have to ask how a lens could be enlarging a speck on a slide if it is not reflecting or projecting it. Kenneth Smith’s comment above, while admittedly detached from its context, does appear to be hinting that the scientists needed to come up with something that resolved the problem of low magnification, although they may also have been attempting at the same time to undermine the idea of high magnification. However, I assume the essay will be more authoritative once I have included a section on electron microscopes.
The following sections of the introduction were first written in 2016 but revised recently to include more discussion of standard texts (so far, chapters in Schaum’s College Physics). The This section following the introduction on microscopes was written in 2017 and 2018 and the essays on cancer and HIV following that were first written in late 2013 but have been amended since then, although the sections on microscopes in those essays have not been modified recently. The advertising copy that follows the two essays was for the second of two advertisements that I took out in the autumn of 2016 in a local paper while on holiday in the United States. I
To view a 'cell' on a microscope slide, high magnifications are said to be needed, for example, 10,000 times to view an HIV cell, so that in theory we would then be looking for abnormalities in a sample on a slide that had been magnified so that its image measured 600 metres by 200 metres. On the other hand, the average cell, according to the Oxford Dictionary of Science, is said to be typically 0.1mm to 0.01 mm, so we would expect be able to see these cells clearly at the highest magnifications of the Celestron and Apex Learner, which magnify 600 to 800 times, so that typical cells would then measure 6 to 80 mm; according to Sadava et al in the Life encyclopaedia of science, the smallest object the eye can see is about 0.2 mm.
However, Sadava et al then state that ‘most cells are much smaller than 200 um’, ie, 0.2 mm, 1um, or a micrometre, representing 1 metre divided by 10 to the 8th, in length, with cell measurement usually being given in volume and ranging according to Life Science from 1 to 1000 cubic micrometres (um3). The fact that um expresses a fraction of a metre rather than a millimetre or even a centimetre might be thought to convey a smaller size than the cell actually is since 1 um is equal to one millionth of a metre but only one thousandth of a millimetre. Although the largest cell would still magnify to 6 - 8 mm, the smallest, at 1 um, or .001 mm would only magnify to .6 to .8 mm, which would be visible, but not so that we would be able to distinguish its parts. However, Sadava et al are perhaps attempting to convey the idea that we might not need an especially powerful microscope when they state that, ‘Most human cells are miniscule. About 2,000 human skin cells lined up in a row would fit across this page.’
However, with the more powerful electron microscopes, said to be reliant on beams of electrons rather than light, we can apparently obtain a clear image of the electron itself moving at 2,200 kilometres a second and magnifications of up to 7 million times. However, whether or not we would be able to view fast moving or stationary images of electrons at very high magnification with beams of electrons and magnetic coils, consideration of the construction of the microscope, and of what we see when different slides are placed on the microscope stage, lead us to the view that it is not designed to see what is on the slide at the high magnifications, that we are not seeing a direct image, and that clear images at high magnifications may not being constructed within the microscope itself. Reading of standard tests and observation suggest that the diagnosis of disease based on histopathology, the analysis of cells under a microscope, is mistaken, whether or not it is also always fraudulent.
II
All magnification might be thought to result distortion because we are stretching an object and so would expect a paler version rather than one showing more underlying features. Also, when the eye magnifies it does so because it is not viewing the object at the correct distance so that magnification is accompanied by blurring. Enlargement is said to make it easier to separate objects or parts of an object that the eye has difficulty in doing otherwise because they are too close, or in other words, it increases ‘resolution’. However, again we might expect resolution, to be at the expense clarity.
With slide projection, an image is projected forward through a lens, and the size of the object is determined by the angle at which light is projected and the rim of the projector and the position of the screen onto which it is projected. The quality of the image is determined by whether or not the lens is focused correctly and how well it is illuminated but an enlarged image is always less clear than that of the slide itself held under the light. Nevertheless, we can sometimes obtain very sharp images. I had thought this might be possible up to the size of the original object, although the limiting factor with my own slide projector I assume to be the strength of the light. The clarity of the image may be due to the fact that we are enlarging a reduced image or it may be because we are enlarging over a longer distance but also with a smaller angle, although this will need further consideration and reading of standard texts. What I have thought recently is that I have perhaps misunderstood the distinction between a real and ‘constructed’ image, in the sense that an image projected onto screen is in a sense constructed, although another sense in which the image might be constructed would be if there were no mechanical link between the object and the image.
Although the electron microscope displays an image projected onto a computer screen, the traditional optical microscope does not but instead apparently allows direct observation of the object directly beneath the final microscope lens. With projection we can see the image increase in size, assuming light travels far enough, as we move the screen away from the projector. However, with the microscope, which is usually made up of two magnifying lenses, we are apparently viewing an object at the position it is located and we increase magnification by altering, or turning, the lenses rather than by moving the position of the object or eye. We cannot see what is happening as easily with magnifying glasses as we can with a slide projector in the sense that there is not an indefinite increase in enlargement as we lift the glass, and the image usually changes in character as well as size, for example, with multiple or upside down images and colour distortions.
Also, with magnifying glasses the limits to clear lens magnification seem to be surprisingly low. The lenses I have acquired do not magnify more than three times and although magnification increases with a second lens, this seems to be more apparent with lenses of lower magnification, so that the highest magnification I obtained with two lenses also seems to be not much more than three times. Neither does a mirror held between the lenses appear to add significantly to enlargement. It was while using a magnifying glass - in fact the magnifying lens of the Sunagor MagnaScope - that I realised that what I was seeing with magnification was not the object itself but a reflected image. When I lifted the glass away from a piece of paper tI realised the image now looked like a reflected one. this finding is supported by inconsistencies in the standard account of what normally happens during refraction and what takes place in the magnifying lens, as will be discussed below.
Normally, we would expect refraction to bend light entering the lens at an angle - assuming it does so - towards the ‘normal’, ie, to travel at a reduced angle through the denser material because the refractive index (the speed of light in a vacuum over the speed of light in the material) is higher in the lens than in air. However, according to Schaum’s College Physics, for example, ‘When a ray passes through a lens it refracts or “bends” at each interface [as drawn in Fig.38-1 in Schaum’s College Physics]. When dealing with thin lenses all of the bending can, for simplicity, be assumed to occur along a vertical plane running down the middle of the lens [(see Fig. 38-2)].’ As well as not being sure why the chapter is called Thin Lenses, the idea that with a thicker lens there would be convergence, according to the diagram, as soon as light enters the lens but with a thinner it begins in the middle seems inexplicable, so that I would assume it is intended to be so. Also, the angle of transmission, or refraction (the angle in the lens, the angle reaching the lens being the angle of incidence) appears from the accompanying diagrams to be a function of the whether it is concave or convex and where in the lens it enters, so that, for instance, light passing through the centre of the lens continues at the same angle. This is despite the author saying at the beginning of the short chapter, ‘This of course assumes the lens is made of a material whose index of refraction is greater than that of the surrounding medium’, which would mean that the angle of transmission / refraction should bend towards the normal, or the line at right angles to the lens, aside from the effect of convexity or concavity. However, the text and diagram following this statement appear to make no such assumption. Reflection off the face of the lens is, however, shown in the diagrams, which might be intended to imply both the difficult of direct viewing and the role of reflection onto whichever surface.
Instead, the divergence or convergence from a concave or convex lens also appears to take no account of the difference in refractive index as the light leaves the lens, in addition to changes in the angle within the lens being determined, according to Schaum’s, by the shape of the lens rather than its material. Also, in the chapter on Thin Lenses, Hecht shows us the different focal points for convex and concave lenses, the first in front of the lens, a ‘real’ focal point, the second behind it, a ‘virtual’ focal point; although the second describes where diverging lines would in theory meet, it does not describe, according to any theory of direct viewing, what we might focus on beyond a concave lens and might instead be hinting at what takes place within the lens, whose interior shape is concave, and therefore where we are viewing the image. In fact, while the text and diagrams may describe what is taking place within a magnifying lens and slide projector, the microscope is usually illuminated so that light passes the other way through the microscope lens, ie, away from rather than towards the object on the slide, so that consideration of how light leaves the lens is beside the point, or the ‘real focus’ may be intended to imply that we are viewing the image inside the microscope rather than viewing the actual object, while the broken lines to the ‘virtual focus’ might or might not be hinting at the difficulty of direct viewing and the necessity of a virtual image.
In fact, the calculation for object and image relation that Hecht presents before the lensmaker’s equation implies that the image is contained in the lens:
1/So + 1/Si = 1/F
where So is the object distance from the lens and Si is the image distance from the lens and F is the focal length of the lens: eg, ⅓ + 0 = ⅓ on the assumption that the object must be at the correct focal length from the lens.
However, after accounting for the position of the focal point of the converging and diverging rays, Schaum then introduces the ‘lensmaker’s equation’, which does apparently take account of the refractive index.
1/f = (n-1) (1/R1 - 1/R2)
where n is the refractive index of the lens material and R1 and R2 are the radii of curvature of the two lens surfaces, and now 1/f is equal to a lens power of m to the -1 in ‘diopters’. What Hecht means by ‘two lens surfaces’ is not made explicit but I assume he means the two surfaces where light in theory first enters and then leaves a lens that is convex on both sides.
‘This equation holds for all types of thin lenses’ also appears imprecise. However, according to Schaum the refractive index of glass is about c / c / 1.5, so that n - 1 = about 0.5. According to Schaum’s, “A radius of curvature, R, is positive when its center of curvature lies to the right of the surface, and negative when its center of curvature lies to the left of the surface. For a positive lens power, ie, m1, R1 would refer to the converging (convex) lens and R2 to the diverging (concave) lens. However, 1/f would also then be smaller for greater values of R1, that is, with thicker lenses. Also, below the lensmaker’s equation, Schaum’s gives us another equation for 1/f, which is to add 1 over the focal length of both lenses when in close contact:
1/f = 1/f1 + 1/f2
Unlike in the lensmaker’s equation we now have two lenses and two fractions with positive values. First of all, for a more powerful lens we would expect f to be less than, not greater than, f1 and f2, although this may be a point about lenses in close contact, and that compounding , where we would expect multiplication of lens power, will be discussed in the next chapter on Optical Instruments. Second, because both f1 and f2 are positive, 1/f would give a negative value for lens power, ie, m-1.
Schaum’s uses the term ‘propagating’ synonymously for travelling through the lens and one wonders if the term is not hinting instead at ‘propaganda’. And Schaum, referring to one of a series of figures says, ‘Notice that a ray heading for the center (C ) of a thin lens passes straight through it unbent’. So that, despite what we are told about the refractive indices of light travelling through different materials, and despite the fact that light bends away from the ‘normal’ in a concave lens and at the ‘microscopic level’, there is apparently no refractive effect of light travelling at an angle through the centre of a lens. That we are able to view relatively clear images when using lenses might support the idea of an unbending convergence and divergence of all rays through the centre of the lens but while this might be one explanation of what is taking place it is not necessarily the correct one and requires further consideration. In fact, the calculation for object and image relation that Hecht presents before the lensmaker’s equation also implies that the image is contained in the lens:
1/So + 1/Si = 1/F
where So is the object distance from the lens and Si is the image distance from the lens and F is the focal length of the lens: eg, ⅓ + 0 = ⅓ on the assumption that the object must be at the correct focal length from the lens.
In the chapter on Optical Instruments, the obvious point is, I think, implied that all magnification is distorted because we are viewing something at a closer than normal focal point.
“Farsighted persons can see distinctly only objects that are far from the eye; nearsighted persons can see distinctly only objects that are close to the eye. [Italics in Schaum’s] …
A Magnifying Glass is a converging lens used so that it forms an erect, enlarged, virtual image of an object placed inside its focal point (i.e., at a distance less than one focal length from the lens.”
The following paragraph presents the equation for magnification, which is the nearest point at which one can view clear (dn) over the focal length of the lens (f) + 1, unless ‘if the image is at infinity, for relaxed viewing’, the equation is simply dn/f. I had already thought about an earlier comment in another book that the angle of aperture could not be greater ‘of course’ than 180 degrees, and had assumed the reference was to the fact that at 180 degrees the image would be infinity; here Hecht is talking about the image being at infinity rather than at the distance for clear viewing, which I assume may be the same thing in reverse, or blurring because, I would think, from the illuminated object rather than from the convex lens, although this will need further thought.
The equation for compounding of magnification is as follows:
MA = (dn/fE + 1) (siO/fO - 1)
where now MA is the ‘angular’ magnification, and is ‘the ratio of the respective angles subtended by the images on the retina with and without the instrument in place’; si0 is the distance from the objective lens to the ‘intermediate’ image it forms; and E and O refer to the eyepiece and objective lenses. Schaum’s states that the equation holds when the final image is at the near point, dn = 25 cm.
‘This equation holds when the final image is at the near point, dn = 25 cm.
Although with the Prinz I make the point following the introduction that the object appears to be at the normal focal point, Schaum’s calculation now seems to be assuming the image should be at the near point and not before it.
Otherwise, the first part of the equation seems to make sense, in that it will have a higher value for stronger lenses, assuming that stronger lenses do have a closer focal point, which from observation they appear to, as would sio over smaller values for fo. My initial observation would be, and this will need further thought, is that I assume, first, that dn is taken to be a constant since we are not moving our eyes nearer or closer as with a magnifying lens. Second, although siO looks like being the same as fO, from looking further up the page , I assume instead that Hecht instead means for siO the distance between the final lens and the image formed on the retina (and one thinks might even be a reference to eye damage). At the beginning of the paragraph on the near, or normal focal, point, he has said:
“The Eye uses a variable-focus lens to form an image on the retina at the rear of the eye.”
The value of SiO over fO would also increase for smaller focal lengths.
While struggling to understand the significance of the two values, dn and siO, I see that below it Hecht writes for the telescope:
“A Telescope that has an objective lens (or mirror) with a focal length, fO, and an eyepiece with focal length, fE, produces a magnification MA = - fO/FE.”
Here, as well as wondering what has happened to viewing without the lenses and the negative value, we find out that one of the lenses (as I observed) is a mirror but Hecht names it the objective lens and the mirror is facing us if we look into the telescope cylinder.
However, while I am sure that the process taking place is reflection, or at least that we are not viewing the object directly if we wish to call it something else, that we are instead viewing a ‘captured’ image, I have myself struggled to understand what exactly is taking place within the lens and at least need to rethink my previous assumption that the image is normally reflected as a significant ratio from the object onto the first face of the lens, nor is it usually onto a mirror, and instead assume that most enlargement takes place within the lens itself, which is supported by the tunnelling one sees with thicker lenses, although this would not support the idea of particularly high magnification, and illumination sources are themselves convex on, for example, the Apex Learner. One difference between viewing an image in a lens and projecting onto a screen that will require further thought is that the image on a screen seems more ‘objective’ in the sense that it does not change according to where we are viewing it from whereas a reflected image, whether in a mirror or a lens, ought to. However, neither did observation of an image projected partly onto an opaque surface and partly onto a mirror support the idea that the reflective surface aided ‘resolution’, which is consistent with reduced clarity with thicker lenses although one might have expected greater clarity with projection onto a mirror.
III
When using a traditional microscope with two lenses, the 'scope' for enlargement might appear to be lower, restricted by the fact that we are reflecting (not refracting) and projecting an image backwards through usually a very small aperture onto small glass lenses within a dark cylinder that may not be the optimal length given the diameters of the lenses and the precision of the illumination, so that our ability to view all parts of the slide with ease, as well as to magnify and view the objects, appears to be no better than with magnifying glasses even if the microscope were to contain mirrors as does the telescope. Artificial illumination does not produce a clearer or more complex image than that obtained with natural lighting at stated magnifications of around 40 times, while some of the complexity we see at higher magnifications with artificial lighting are instead light distortion or light patches.
With both light and lens projection (ie, projection of light within or from the lens), even at low magnification there will be distortions because the angle of projection or reflection decreases as it approaches the centre, with enlargement greatest at the edges of the image. For example, we can sometimes see this effect in a photo of ourselves if the face looks too wide. As well as the image dissipating the further from the light source because of insufficient light, there will also be distortion, blurring and loss of visibility as the angle of projection from the lens produces a larger image.
The standard microscope itself is not constructed to maximise possible magnification: the slide is very close to the microscope, the final aperture is very small, as are the objective lens tubes, so that we would theoretically, with the more powerful of the two lenses in the Prinz 2801 microscope for example, only be able to obtain an image of what is on the slide beyond the microscope, if it were sufficiently, but not over, illuminated, enlarged by around 3.3 times, which is consistent with what we find when using magnifying glasses. A speck would not normally be magnified to the width of the lens: a larger area is reflected - in the same we do not normally see a speck from a photographic slide projected onto a screen nor only a speck reflected in a mirror - with the microscopes I have had access to, and it seems likely that the precision required would be both unobtainable and unnecessary if we could instead use a larger lens, such as one the size of a normal magnifying glass. However, even if a much smaller area were the subject of the projection or reflection with sufficiently guided illumination, clarity would in any case be lost rather than resolution increased by enlargement.
IV
Magnification - defined by Dorland's Medical Dictionary as "apparent increase in size under the microscope" -occurs to a limited extent when we bring an object closer or look into an empty cylinder because we focus on a smaller area than usual. More significant enlargement is the result either of light projection, as with a slide projector, or reflection within a magnifying lens. The traditional microscope, which uses light and lenses, is usually described as containing two lenses, an ocular lens, below the eyepiece, and an objective lens, at the end of each of the three objective lens tubes lower down the microscope, so that enlargement is the result of magnification by the first lens and compounding by the second lens. It is useful, in attempting to judge the limits of microscope magnification, to observe what we can see with the eye alone under strong light conditions, what we can see with one or more magnifying glasses and, given its role in the telescope, lenses and a mirror, and judge this against both the claims made for the microscope and the apparent size of the image we see.
With a magnifying lens, if we hold it right up to the page we are reading, there seems to be no enlargement of the letters behind it. However, as we raise the lens, the letters grow bigger as they are reflected within the lens. That this is not simply light projection from the object is indicated by the fact that the size of the image varies according to which lens we use.
Although the standard account of magnification is that we view the object directly through the lens, observation of what happens as we use a magnifying lens suggests that we are instead viewing a reflected image. The standard explanation of enlargement would be that we see an image refracted, that is, stretched in some way by the lens, or by light entering and leaving the lens, so that the object gets bigger the further from the lens, as a slide projected gets larger the further it is from the projector. Yet, unlike with light projection we are not projecting an image of the object beyond the object itself: instead we are apparently viewing the enlarged object at the same place it is situated.
Instead, as we lift, for example, a magnifying glass from a page of writing, we appear to see a reflected image. Enlargement would then be determined by the angle at which light is directed from the object to the lens, but not only this or we would not obtain larger images with thicker lenses. Since the material of the lens would be thought to reduce rather than increase the angle of enlargement if the mechanism were refraction, what I assume is instead happening is that the image is re-reflected within the lens, the material of the lens influencing visibility but enlarging an image that has been reflected onto only part of the first face of the lens or partially re-reflecting onto the second face. The fact that we are viewing the front rather than the back of the lens would seem to be supported by the tunnelling effect we see if we continue to lift a magnifying lens or our eyes from the lens or sometimes with more than one glass. The size of the image is influenced by the distance of the slide or other object from the microscope, by the size of the aperture and lenses, the length of the tube and cylinder and whether or not partial re-reflection takes place according to the angle of projection. The object is reflected within a lens that behaves as a two way mirror, in the sense that we are viewing the reflection from behind and therefore the image will not usually be viewed back to front, although as we manipulate the slide we may see it move in the opposite direction either horizontally or horizontally and vertically, I assume because of the angle of reflection, as will be discussed later. Although reflection is likely to increase rather than decrease the scope for enlargement in the sense that it does not rely on a large angle of refraction within dense glass, this is already a radically different explanation of why enlargement occurs. The account of what happens within the lens does not necessarily undermine the standard view of convexity and concavity, if, if there is convergence inside the lens, but the fact that the lens is convex (although in the case of the microscope sometimes behind a plane aperture, which is also not optimal) supports reflection rather than refraction, since the lens reflects a smaller area initially as it enlarges but then behaves a concave lens as we continue to lift it. That this is true can be observed most effectively by changing the lens to one of a higher magnification and noting that a smaller area is illuminated.
V
With the magnifying lens, as we lift it still further away, the image we see starts to become smaller. Before this happens, there will be distortion, for example, as the edge of the object appears more stretched and blurred than the part of it nearer to the centre of our vision, which is taking place as we try to enlarge more than three times. Before returning to normal size when the lens becomes so close that we view as though there is no lens, the image becomes smaller, which may be because the ratio of projection is such that the point of convergence is after rather than before the centre of the lens, but we can also observe the lens focusing on a larger not smaller area as we lift it, as though it were acting as a concave lens.
If instead of converging to the point of curvature within the lens, the light or image instead diverged from the concave interior or, as does not seem plausible, there was not a series of reflections but instead reflection from one face of the lens to the other, the extent of magnification would still be limited by the width of the lens, while magnification of the smallest objects on the slide, a speck, would be limited not only by its distance from the lens, and the inability to illuminate sufficiently precisely, but also by its size relative to our field of vision. Lens magnification is relatively constant. In other words, we are viewing a smaller area than we would without the glass or if the glass is held too near or far from the area. Although some parts of the image will be magnified more than others, so that the image appears stretched and blurred at the edges, the extent of magnification is determined by the relative sizes, or ratio, of the whole area we are viewing to that of the lens, as well as by the character of the lens, so that we are not, for example, magnifying a speck simply by the ratio of the size of the speck to the diameter of the lens.
Although it might be theoretically possible to expand a speck to the width of the lens with light projection if a light were placed under a translucent object, the actual limits of projection would be determined not only by the strength of the light but by the angle of convergence of the light, which if it is not encased, will not converge (or diverge) sufficiently to produce, say, a ratio of one to sixty (the usual magnification claimed for the objective lens of a traditional microscope), especially given that it is leaving a mirror placed at around 45 degrees, so that some diffusion occurs.
Although a thicker lens potentially increases the size of the image for a given angle of reflection within the lens, with lens magnification we get distortions such as the tunnel effect, and loss of visibility of the object beyond it, as we focus increasingly on the lens or lenses rather than the object beyond them, while blurring and loss of visibility occur also as a result of over or under illumination. Also, moving the lens further from the object leads to back to front or upside down images, since as we raise the magnifying glass and focal length returns to normal, objects no longer in our direct vision are reflected at an angle. In other words, in the same way as an object to the right of our vision will appear on the left if we look through a lens (which is in itself relevant), so whole objects will be rotated if their edges are to the left and right of our direct vision. Another distortion is multiple images, I assume because smaller displaced images are bounced, or reflected, onto other parts of the lens. Finally, as the thickness of the lens increases, whether or not there are distortions, the image will become less clear the greater the distance from one side of the lens to the other, especially if it not projected by a strong light onto the lens and especially for objects closer to the lens or lenses.
However, whether or not high magnifications can be achieved or produce clear images in theory, the microscope is not constructed to view the slide at the highest magnification possible. On the microscope I first acquired as a child, a Prinz 2801 Microscope Outfit, the objective lens tube containing the lens with the highest magnification is approximately 1.0 cm in diameter, and 1.5 cm in height, and the plane, rather than convex, aperture is around 3 mm in diameter and the lens behind it claims a magnification of 60x. The slide, placed on an aperture on a microscope stage of about 5 mm in diameter, is only about 5 mm below the lens aperture and the part of the mirror from which light would be projected about 30 mm below this. This would mean that whether reflection is via direct projection or lens magnification, the limit would seem to be 3 to 10, ie, the ratio of the aperture to the width of the objective lens tube, since a smaller object – ie, one of less than 3 mm in diameter – will be projected or reflected at a proportionally smaller angle, since the angle of light entering the aperture, which determines the enlargement, will remain the same whatever the size of the object to be magnified. The microscope is not built to be taken apart and it can seem as though one is looking into a hollow cylinder rather than one containing two or even one lenses. Nevertheless, the character of the image one sometimes sees under certain illuminations - the one I saw initially with the Prinz - does suggest that the microscope contains a lens or lenses of some sort, and in fact with the Apex Learner I acquired later the ocular lenses are detachable.
The limit imposed by the diameter of the aperture and lenses and its proximity to the slide is in addition to the problem of viewing material on the slide whether or not it is magnified, such as the fact that the aperture only views a small portion of the slide and that we are attempting to view a slide beyond two small apertures and a dimly lit cylinder and lens tube. At high magnification with a traditional microscope we would only view a portion of the sample on the slide at any one time even with a larger aperture. However, we would have difficulty seeing anything whatsoever on the slide directly when looking into a small eyepiece and ocular lens, if there is one, through a dimly lit tube - greater illumination leads to blurring on the screen - and then through a second lens, if there were one, and through a tiny aperture to a slide beyond it, even if the slide is brightly illuminated by artificial lighting or natural lighting and an external mirror. We can see no image with only natural lighting when there is no external mirror, as is the case with the Apex Learner, which might support the idea that with sufficient illumination we can view directly, despite the dark cylinder or because of it. However, although viewing an object through a cylinder aids focus on the relatively brighter object beyond it and illumination of the lower end of the tube itself is increased by the addition of a final lens, we are straining to see an image, whether reflected or direct, when we add a lens on top: either the tube is insufficiently illuminated to see a clear object or we see a blur if the final lens is over illuminated.
A higher degree of magnification could be achieved in theory by projecting the image of the objects we wish to view forward, but setting aside the question of what clarity we might expect if a slide were projected onto a screen of 200 x 600 metres (at a magnification of 10,000 times), the microscope is not constructed to optimise light projection since we are not, with the traditional microscope, viewing an image beyond the the slide.
The fact that when we look into a microscope we do, however, see a relatively clear and detailed image indicates that we are unlikely to be viewing the object directly and may also not be seeing an image of an object reflected onto two slides. In the Prinz, I initially saw the same basic image whichever slide was present on the microscope stage. Although this was the case over a year or two, admittedly with relatively few observations, before I acquired my second microscope and is as likely to be the result of my having not found the correct focal adjustment as now seeing a remotely constructed image, what I did see is of interest because it is also sometimes present with higher stated magnifications as though it is the result of higher magnification when it is in fact likely to be an image of the lenses themselves, although this fact at least supports the idea of some sort of mechanical reproduction of the image rather than remote accessing. However, although my observations are included below, it may be that the initial absence of an image of what was beyond the microscope was a ‘red herring’.
What I initially saw with the Prinz was an image of relatively fixed shadowy and translucent objects on a circular screen at the end of a cylinder, as well as translucent and moving objects in layers further up the microscope. Both images included ribbon-like objects of a similar length and width and with similar knots in them, although there were dark spots present on the image on the screen. These images remained whether or not the slide is present.
The translucent and moving images higher up the microscope are the lenses of our own eye (ie, those of the microscopist). We know this because the image is the same as we see when looking into bright light and because the image shifts as we shift our eyes. However, the image on the screen further down the microscope, and sometimes of the ocular lens, although also resembling the lens of the eye, was fixed. In fact, when I turned the microscope upside down, I saw just above the objective lenses what looked like the small orange irises of a bird facing into the microscope, supporting the ‘red herring’ idea. Because the image on the screen resembled the lenses of our own eye, it seemed plausible that the object we are viewing is in fact the lens of an eye. The fact that only the position of the image relative to fixed sides changes when the lenses are rotated suggests projection forward, rather than backwards from different objects, as does the opaque look to the screen (we seem to see nothing beyond it). It therefore seemed plausible that a fourth object, the lens of an eye or an image of one (although parts of it appear shadowy, the ribbons and knots are translucent if there is sufficient illumination), is placed higher up the microscope, projected onto an opaque screen further down the microscope. Why would this be done when we can see a clear image of what is beyond the microscope with the correct focus and illumination? Perhaps to satisfy or frustrate the amateur user about the nature of high magnification in the absence of a revealed image.
True magnification in the microscope, in other words if one is not viewing a constructed image, would occurs partly as a result of a reduction in focal length as one looks into the relatively short lens tube of the microscope, where the effect of the reduction in focal length is to make the cylinder appear longer than it is and the circular screen larger than the diameter of the lens tube. The cylindrical shape enables us to focus on the objects on the screen, which would otherwise be blurred because they are too close. The focal adjustment may vary focus for different users but also according to the position of the microscope in different lights. The objective lens aperture lets in light when the microscope is lit by the mirror or battery lighting. Looking closely into the eyepiece also reveals that there are likely to be an inner and outer tube since rotation of the eyepiece results in rotation of the edges of the screen whereas rotation of the focal adjustment results in a rotation of the image on the screen. This is implied rather than shown in drawings of the optical system of the microscope. Rotating the focal adjustment does not alter the size of the objects but does change their position and allow us to see more or less of them, indicating that more or less light is let in below the screen on which the visible objects spiral as the adjustment is turned. When the Prinz microscope was lit from the mirror, an opaque object would block visibility so that nothing was seen on the circular microscope screen. A translucent sample would not alter the image on the screen. However, when a stain was put on a slide, it would appear as an undifferentiated wash over the microscope screen. This remained true when recently I was able to view a clear image of, for instance, a piece of lens wrapping without a stain over it, supporting the idea that the slide stain does not improve ‘resolution’. Sometimes a slide would produce a complex image, although not the colourful tile pattern that I saw once when doing an observation externally and have seen recently, that led me to think that I might be viewing something at a higher magnification. Instead, I decided I was viewing a pattern of light and shade from objects of varying opacities at relatively low magnifications. Light would shine through some parts or objects on the slide but not others, and to a differing extent, so that there were shades of dark and light. The vivid variation I saw when using the microscope once in a cafe I thought at the time to be the result of the tiling effect on a ceiling light but was very similar to one I obtained recently, I think of a piece of lens wrapper, and I think using the Celestron rather than the Prinz.
The limits of magnification may be being hinted at in this quote from a textbook with a 1931 publication date: "If well corrected lenses are used, the magnifying power of the microscope should be at least that necessary to reveal the finest details resolvable by the objective. For the normal eye, this is equivalent to about 500 to 700 times the numerical aperture of the objective." This may be hinting both at the difficulty of viewing the image and the fact that much of the complexity revealed can be seen by the eye alone without magnification.
Diagnosed diseases cause people fall ill or die, as well as restrict lives in other ways, for example, by affecting choices about reproduction, not because they are real diseases but because of factors such as fear and fatalism, insufficient or poor nutrition or a restricted diet, including the denial of food and water in hospitals, extreme variations in temperature, gassing or increasing ‘pressure’ in and out of hospitals, and probably the premature pronouncement of death. Once one accepts the idea that diseases may be fictitious, one can think of other arguments and make other observations to support this apart from the implausibility that we are viewing the cell under the microscope, such as, for instance, the fact that the link between DNA and mutation seems to be asserted rather than explained and to have no foundation in the philosophy of science, since the ontological incompatibility of the abstract and the physical is not resolved (there is not, nor can there be, a mechanism by which one influences the other). However, while I have attempted to address other aspects of disease in two later essays, what follows is first a further discussion of the microscope, written after some of the points in the introduction and so contain a certain amount of repetition that I hope will reinforce the argument. I begin with what might seem another introduction, followed by recent observations, followed by points that broadly follow from them although many were made after observing magnifying glasses, and those observations I did not write up - apart from estimating actual magnification for each lens - although I intend to repeat them and include those observations in a later draft.
- On whether microscopes magnify sufficiently, or achieve sufficient resolution, to view cells and diagnose diseases.
Although cancer was apparently diagnosed before the invention of the electron microscope, diagnosis since at least the 1960s has relied upon it. I have had no access to an electron microscope but was initially sceptical that the traditional light and lens microscope viewed what was beyond the lens or, if it did, that it could achieve anything like the higher magnifications claimed. I then saw that high magnification was apparently possible with a traditional microscope, as much as 600x with the Apex Learner. However, because of distortions at low magnification with magnifying lenses I thought that the light microscope must then be an electron microscope, neither microscope in the sense of replacing light with beams of electrons, but because they depend upon remote (for example, satellite) accessing and the construction or modification of an image unless, in the case of the light microscope, a kaleidoscopic effect is produced mechanically within the microscope, which, from observation over time, may be less likely.
I have perhaps not yet proved that I am not seeing a significantly enlarged image of the object itself rather than a reconstruction whether mechanical or remotely constructed with the traditional ‘light’ microscope, and that the complexity and focus is not the result of better illumination, any remaining doubt apparently supported by the greater complexity we see if we hold an undifferentiated object, such as a lens wrapper, up to a strong light. However, while holding an object up to the light may reveal a large number of small parts - or particles - it is not plausible that we would view these clearly magnified 800x in length (the highest magnification stated for the Apex Learner) or that the microscope is designed to achieve it (if, for example, one compares it to a slide projector). The fact that the magnifications differ fairly substantially from those claimed by the manufacturer with the three microscopes I have that ‘work’; that we are in any case unable to measure enlargement precisely because we are estimating the size of the screen on which we view the image; that some of the complexity and magnification appears, in fact, to be light distortion or patches; that it appears to be difficult to obtain consistency in terms of the size of the image and its individual parts (and, more recently, in terms of the quality of the image); that in fact the similarity in structure between what we see with the eye alone under a strong light and the image we apparently obtain at high magnification with the microscope suggests we are not viewing an underlying structure; that the measurement of magnification for diagnostic purposes is in length only so that the highest magnification the light microscope is capable of is nevertheless insufficient to diagnose disease; the implausibility of electron beams and other mistakes in explanations of magnification, including the fact that we are not viewing the object directly with lens magnification but, from observation and the fact that as we increase magnification the image on the slide becomes more, not less, difficult to find, are seeing a reflected image; as well as the less than optimal design of the microscope, including the tiny objective lenses; has encouraged me to think I am right that microscopes do not view cells.
The following is based on observations using, first, the Prinz 2801 (a standard two-lens optical microscope that could initially be lit by either from the mirror or a battery but now can only be lit from the mirror), second, the Sunagor MagnaScope (a two-part optical microscope consisting of a zoom and magnifier with a funnel to direct light), third, the Apex Learner (an optical microscope, that can be lit either from above or below but not by natural light ), and, most recently, the Celestron 600x (another two-lens microscope, that can be lit either from above or below or from the mirror). Each of these are traditional optical, or light, microscopes that depend on battery or mains, mirror or natural lighting and magnifying lenses to achieve enlargement. Until recently I had underestimated what could be seen using the light microscope because I had depended only upon the Prinz 2801 and Sunagor MagnaScope, both of which relied on natural lighting after damage, one of which could apparently not see beyond the microscope, even if it showed quite complicated and ‘cell’ like structures on the screen, and the other which could, but with magnification of what looks like only around 15 times in length with natural lighting.
However, the Apex Learner, which is illuminated either from above or below the slide, achieves surprisingly high magnifications. With the Apex Learner I appear to see a two-millimetre wide piece of transparent bronze film – the ocular lens wrappings - magnified so far by as much as 600 times –360,000 times if we think of magnification instead in terms of area, although pathologists do not. However, it is impossible to assess the actual magnification since we are judging the width of the screen we see within the cylinder rather than an image projected onto a screen (and for this reason too it can be difficult to obtain consistent results). However, magnification appears to reveal structures that cannot be seen by the eye alone, although with the object observed (the bronze-coloured wrapper) these appear to be a pattern of opaque and translucent (reversed if we light from below rather than above) rather than an underlying structure. I have only recently acquired the Celestron but nothing I have observed so far contradicts my findings with the other microscopes.
When I obtained the higher magnifications I did not doubt that microscopes did not diagnose disease, which I have been certain of since guessing in late 2012, but was not sure how to account for it. A National Geographic telescope I own contains two mirrors, but whether or not there are mirrors inside the microscope – and I have so far been unable to take any of them apart - these would not, from observation of what one sees , for example, if one places a mirror between two magnifying glasses, add significantly to enlargement. Instead, I wondered first why it would have been necessary to have invented the implausible-sounding electron microscope rather than continue to use the light microscope for diagnosis of disease unless it were to discourage amateurs and then whether what was being implied by the name was not in fact beams of electrons providing illumination but that all microscopes are electron microscopes in the sense that they resemble other electronic communications devices: the outline of the image or the object or stain itself is detected, enlarged and filled with a constructed complex structure.
The sections following observations from the Apex Learner are sceptical with respect to traditional accounts of magnification but have been influenced by what I take to be hints in these as well as other literature. There appear to be mistakes in standard accounts of magnification. For instance, we are viewing a reflected image rather than the object itself, so that what is actually happening within the lens is re-reflection, determined by the character of the lens and illumination. The highest magnifications I have obtained are also lower than those claimed by the manufacturer, even if surprisingly high. The highest magnification possible with the light microscope, according to the department of biological sciences at Leeds University is 1400 times, less than that needed for cell pathology. However, given that this is almost 2 million times if one thinks of enlargement in terms of area, one wonders if the definition of magnification in terms of length was not intended to prevent self-analysis of cells.
Accounts of the electron microscope, on the other hand, assert that higher magnification and resolution, or clarity, can be obtained with electron beams and magnets, with magnifications of as much as 7 million times. [encyclopedia of biology] The mechanisms chosen, electron beams and magnetic coils, may be intended to undermine criticism of diagnosis, as might also the subject of light waves, or to encourage it, although the name may also be chosen to imply that what we understand to be a new kind of microscope may instead be remote accessing and construction of the image.
Dorland’s Medical Dictionary defines magnification as “apparent magnification under the microscope”. We can see very small objects – such as mould spores – with the eye alone, while the eye itself can achieve relatively high magnification: the structure of the cornea of the eye is revealed when we squint into light. Also, with limited magnification, especially but not only when using a zoom lens, we can have the impression of significant magnification when, if we actually compare the size of what we are viewing with the size of the object itself, we realise there is little or no actual increase in size: the illusion is created by viewing an object that is further away. Also, with a magnifying glass we may notice that it is the eye itself that is doing the magnification: for example, if we place a lens directly above an object on a page and then hold the lens closer to our eyes, the object will appear larger than when viewed without the lens but so may the lens itself, ie, the object may occupy the same proportion of the lens, although usually it will occupy somewhat more . Nevertheless, the light microscope does also appear to achieve surprisingly high magnification when we compare it to a camera that blurs at limited magnification (from observation, as little as 2 to 5 times, depending upon what we are viewing and certainly more than is possible with the eye alone). Dorland’s phrase may be referring to the fact that it is impossible for the microscopist to measure magnification in the traditional microscope - without taking it apart, which I have not been able to do with the Prinz, Apex Learner or Celestron - because we do not have certainty as to the width of the screen we are viewing, whatever the stated or calculated (ie, taking into account convexity, refraction, positions and size of lenses) magnification of the ocular lenses and the width of the objective lens tubes. Or he may be referring to the character of the electron microscope. Although I have as yet no knowledge of electronics, whether or not the magnetic coil detects the object or its reflection, while any subsequent increase in size is enlargement whether or not it is a reconstruction, the complexity is added, presumably as a result of external accessing and manipulation rather than from inside the microscope, so that we are therefore not viewing an enlarged image of the object on the screen. This would be consistent with the fact that we appear only to achieve relatively limited clear enlargement with light projection and magnifying lenses.
All enlargement using light projection or lenses results in loss of clarity. This is self evident: although we can bring an object into focus that is otherwise too far away or too close to bring clearly, any enlargement will weaken in the sense of blurring the image. With light projection, we may be able to view a slide significantly beyond its size but the image will be less clear than the object at the same size and blurring and loss of visibility occur as the size increases or because of insufficient light at greater distances. The image is dissipated, or becomes less clear, with projection because, as with any enlargement, the space between outlines increases but is also distorted because the angle at which the object is projected is smaller nearer the centre. It disappears when the lines that define objects have disappeared or when the light can no longer reach far enough or is too diffuse. When using a camera we can bring an object closer but often not enlarge without loss of clarity or, much beyond 2 – 5 x (depending on what we are viewing), identify objects. A zoom may allow us to see an object in the distance relatively clearly. However, even without enlargement beyond the actual size, we will see a less clear image than the original because the effect of a glass lens is to reduce rather than increase clarity, which we can observe if we were to move closer to the object and view it at normal focal length, or if we compare the image obtained with a zoom with that obtained if we improve focus by cupping our hands in front of our eyes.
“1. (in optics) A defect in the image formed by a lens or curved mirror.” Entry for ‘abberration’ in Oxford Dictionary of Science, page 1.
Whereas with slide projection, although there is an increasing loss of clarity, the image we see increases in size as we move the object from the slide, with a magnifying lens (or microscope) we are not attempting to project an image beyond its location and there is not an indefinite advantage in placing the object at an increasing distance from the lens although magnification also increases as we remove our eye from the lens as though projection of the object is continuing beyond the lens, although as when we think the objects in a mirror change when we look at it from a different angle but they do not, so the reflected image remains the same whatever our perception of it. This needs further consideration but can in part be explained by the fact that although the lens appears to remain the same size, the size of the screen with a larger number of objects on it appears much larger when our eye is against the eyepiece, although not entirely since the actual size of the individual object on the screen appears greater when viewed from a distance. It is as though the convexity of the eye itself were resulting in a smaller area being reflected onto the eye, the eye itself mimicking the action of the lens and compounding magnification, but this will need further consideration.
With lens magnification, we get blurring, loss of visibility and distortions with enlargement, and the object then becomes smaller as the effect of convexity reverses and recedes. With convex lenses, the distorting effect is also greater than with enlargement from projection alone because the angle of enlargement is determined by curvature as well as illumination. We can see the distorting effect of lenses if we look at ourselves in a slightly curved mirror. Reflection - which the next section explains is what is actually taking place with lens magnification - is usually onto a three dimensional object (the lens) whose surface is different from that of the object being viewed, unless it is a similarly shaped sphere, the distorting effect being greater with greater magnification. The three dimensional nature of the lens also increases relative flattening at the edges. Other distortions include: uneven magnification and distortion of shape, light distortion, the image turning upside down, objects being displaced from left to right or vice versa, multiple images, increasing opacity, a tunnelling effect, and will be considered after discussion of what is occurring when lens magnification takes place, after a summary of observations from the Apex Learner.
“If well corrected lenses are used, the magnifying power of the microscope should be at least that necessary to reveal the finest details resolvable by the objective. For the normal eye, this is equivalent to about 500 to 700 times the numerical aperture of the objective.” [1931] [ie, difficulty viewing image; also point of focal adjustment, ie, not responding to eye and not therefore direct viewing]
Initial observations with the Apex Learner can be viewed in the Appendix but what follows are more recent observations where I obtained clear images approaching the magnifications claimed. My aim has been to establish the extent of magnification, although I intend once I have obtained consistent results to turn to the character of the image. Because one is estimating the size of the screen and objects on it and because of the difficulty of manipulating the image to then measure with accuracy the size of the object (for example, a piece of bronze-coloured translucent film – the wrapping for the lenses - cut first to approximately 1 mm in width and then to 2 mm and placed under a slide casing), I have repeated the observations in an attempt to obtain consistency and to avoid the temptation to underestimate magnification and record true results.
Observations
(i) First, carrying out the observation with a piece of bronze translucent film placed horizontally on the slide, and cut fairly consistently to about 2 mm in width, the size of the width of the foil on a screen appearing to be about 100 mm appeared to be 50 mm, 120 mm and 450 for the three objective lens tubes with a 10x eyepiece lens, supporting magnification of 25x, 60x, and 225x. Manipulation was difficult because the image appeared less differentiated although no less complex than with a previous piece of film (see Appendix 1). Nevertheless, we see within the ribbon thousands of tiny parts, if not completely clearly, resembling something like the map of a city. Of interest in this observation was that I noticed that with illumination from below one saw the image in negative: which is consistent with the structure reflecting patterns of light and opaque. Magnification of 25x, 60x and 225x differed from the stated magnification of 40x, 100x and 400x, and further observations will be undertaken to establish whether the variance is real.
(ii) Carrying out the observation with the same piece of film - 2mm and the 20x eyepiece, the width of the screen appeared to be about 150 mm rather than 200 mm, but it was difficult to make the judgement. With the 4x objective lens tube , the width of the ribbon appeared to be at least 150, which would indicate a magnification of 75x rather than 80 x, an insignificant variance given the difficulty of estimating the width of the screen. With the 10 x objective lens, the width of the piece of film appeared to be about 300 mm, which would indicate a magnification of 150x. With the 40x objective lens, it was not possible to position the lens tube so that the film could be viewed in focus. Instead, I cut another piece of film and placed that on the stage without a slide or casing. Then the width of the magnified object did appear to be about 4x that of the same object (ie, a part of the film, given the difficulty of manipulating and keeping in focus the ribbon when not in a casing – and whether or not the apparently shifting image was or not the result of any sort of external manipulation, this was when I first thought that ‘electron’ microscope might be code for remote viewing of all microscopes). This would indicate a magnification of at least 600x (rather than 800 times). At this highest magnification we see a similar and remarkably complex image with the thousands or tens or hundreds of thousands (repeated observation may establish which) small parts, mostly roundish, some more rectangular, as well as wider lines, larger than at lower magnifications but not obviously otherwise clearer or more complex (apart from the more rectangular shapes being more visible than at lower magnifications) or more numerous. The variance between the magnification obtained with the Apex Learner and what I had seen with magnifying glasses seemed hard to account for. The image is also remarkably still at high magnification. This has led me to believe that although the light and lens are able to detect the shape of the object, the enlargement of the outline is a reconstructed one and the complexity added, so that the Apex Learner must be an ‘electron’ microscope.
(iii) Second, carrying out the observation with a piece of Jaffa cake wrapping 2 mm wide [added subsequently from memory so that I have changed the estimation of magnification that follows; I lost the two bits of film I was working with but think the biscuit wrapping was not 4 mm, which the initial estimation suggested], I found magnification of approximately 2-400x in length or 4-16,000 times in area, which is said to be the magnification needed to view the HIV cell; this was achieved with the 10x ocular lens and 40x objective lens and so may have reached the 400 x claimed. I estimated the screen width to be about 80 mm and the piece of foil to be about 5 times the width of the screen. During this observation I was less convinced than in the previous observation that there was either remote manipulation or even a kaleidoscopic internal adjustment of the image, even though the image was not entirely clear as I moved it. Nor did the stillness of the image seem as suspicious as when I had carried out the observations with the lens wrapper. This then seemed a remarkably clear image given the magnification, even if the increase in magnification of individual structures seemed more apparent than real in terms of the actual increase in length as opposed to area. At 4x I saw the foil cover most of the screen so that the stated increase from 40x to 400x was in fact by about five rather than one hundred times. If I hold the wrapper up to the light I can just see the edge of the wrapping - where I cut it. I can also see cracks in the wrapping and what look like dust particles that may account for some of the complexity. Comparing this with what one sees with a magnifying glass of 1.5 or 2. I can start to see, especially with strong illumination, that the complex structure is discernible, the lens wrapper particularly looking something like a layer of skin. While on the one hand it does not look like, for instance, the creases one can see with the eye alone have been magnified, say, 400 times, I cannot yet say with certainty that I am overestimating the size of the screen or that the complexity is constructed or reproduced, particularly where the width of the foil covers less than the screen. What I can say is that the magnifications claimed are fairly inaccurate, whether length or area, and that this seems odd, given that it does seem to achieve high magnification. and that the resemblance of the image under the microscope to what one sees when holding the film up to strong light may support significant enlargement but not the revelation of an underlying structure.
(iv) Repeating the observation again with the biscuit wrapper and the 20x eyepiece lens, what looked like a 2 mm long crack in the piece of wrapper was magnified to what looked like 40 mm with the 20 x lens and 4x objective (20x) but assuming a somewhat smaller - 60-80 rather than 80-100 than with the 10x lens - rather than larger screen than with the lower strength ocular lens. With the 10 x objective, the same crack was visible bus appeared to take up more than the screen, about 100. The length of the crack appeared to increase around 1.5 times, implying a magnification of between 30x (assuming the lower magnification was correct) and 50x. This is less than the 80 and 200x claimed by the manufacturer, although a lot more if the magnification were based on area, which it seems to be with the Sunagor MagnaScope. Although the stillness of the image is compatible with our seeing the object rather than a construction, neither on the other hand at clear magnification of 50x or 2500 x are we seeing any moving parts. With the 40x lens, interestingly, one can see that the widened edge of the wrapping is, in fact, distortion that, depending on the position of the lens, can be present or absent without loss of clarity of the rest of the image. At this magnification as with the 10x and 40x, what I take to be imperfections and particles on the eyepiece lens, are visible. I am less certain than I am with magnifying lenses that I am seeing a reflected image. However, whether or not the apparent difference between the glasses and the microscope is better lighting, notably in the case of the Apex Learner, from the round shaped light below the microscope stage, although we see more clearly and perhaps in more detail with the stronger of the two lights, the size of the outline is the same whichever of the two lights is used and also with natural lighting, where, however the detail is more difficult to see, which does not support the idea that we are seeing the underlying structure of the cell with the microscope. It also seems unlikely that however bright and guided the lighting that we would be able to see through the cylinder beyond the lenses rather than that the image is somehow captured in the lenses themselves. If it is thought unlikely that we would be able to see so clearly within a lens, the magnified structures or imperfections in the eyepiece lens are clear when the light is on and there is no object present, as is the image we see in a mirror. However, it does seem most likely that better lighting is responsible for the greater complexity rather than that there is an added construction. However, in the next observation I will look more carefully at light distortion - which at the end of the last observation I saw could produce an apparently clear image of a widened edge of the wrapping but which disappeared as I adjusted the focus so that the edge was not all that much wider than when viewed with the eye. I will also try and establish with more certainty that there is no kaleidoscopic effect, or reproduction of parts of the image within the screen.
(v) Repeating the observation with the 20x eyepiece lens and 40 x objective and using a piece of biscuit film wrapping in order to determine the extent of magnification only (ie, less concerned with the structure of the image), I found that the individual parts do not seem to be magnified much beyond 40x. This does not mean that the eyepiece lens is faulty because there is apparently magnification of more than 40x of the width of the film and because one can see compounding by comparing the image of an object with both lenses and by simply looking into the cylinder with no eyepiece lens present. I was surprised by the length of time it took for me to find an image even despite the difficulty of manipulating the film without it being on a slide under a slide case. What I have also noticed before but had not added here is the difficulty at times of distinguishing between the enlarged object and the underlying fixed image on the screen as well as sometimes the cornea of one’s own eye that is also present, although unlike with the Prinz 2801 I am, with the correct focus, obviously seeing a new image, which appears to be that of the object underneath the lens. I have also not yet become adept at manipulating the object lit from below (which it has to be at higher magnifications) so that when moved to the left it appears to move to the right. However, manipulating it by hand - ie, without a slide present - also means that it may be nearer or further from the lens and light so that the size of the object may vary as one moves it, as for instance, the object can disappear when the lens is closest to the microscope stage and also appears to shift significantly as I adjust the focus. However, manipulating it by hand is a problem at the highest magnification with this microscope only.
(vi) In a further observation with a 1 mm piece of the orange-coloured film and the 20x eyepiece lens, an object within the image appeared at about 30 mm on a 120 mm screen (30x) with the 40x and 10 to 15 mm with the 10 x (10x - 15 x)and about 5 mm with the 4 x (5x). However, the object disappears relative to the image as a whole - for instance, one sees a thick and complex edge of the ribbon disappear to a virtually invisible one - as one adjusts the focus, most clearly with the 40x lens, and in fact so do many of the small objects within the ribbon of film, not so much, as we can see with the 40x lens, because of loss of focus but because they are ‘light spots’ that disappear or come into view as one turns the focal adjustment. On a repeated observation it seemed more as though the spots or objects disappeared as I turned the focal adjustment as the object got larger or smaller but even so looking more like one sees in a kaleidoscope than the blurring you get with a camera. I was not able to estimate the width of the object this time because of the problem of manipulation and seeing where and there which width of the ribbon I was viewing.
(vii) Going back to the 40x and 20x the next day, the image was less clear in the sense that the eyepiece lens looked more opaque even after dusting. This is more in line with what I was told in a phone call yesterday that I understood to be with the manufacturer, which was that the image would not be clear at 40x and 20x. Since the light seemed also to be worse for the eye, I did not try again the same day. I was, however, pleased that a mark that I thought had got onto the condenser and might have been confused with an object seemed to have disappeared.
(viii) Repeating the observation with the tape in the same place and with 20x and 40x, I found it more difficult to judge width or identify objects, there being less clarity than the day before yesterday, particularly when I manipulated the film by hand to try to establish width), although I had initially thought there was not the problem of greater opacity with the second of the two 20 x ocular lenses I have (having reordered one, thinking it was not the one I originally had, which I had imagined to be bronze coloured, I now assume having confused the wrapping in my memory with the actual lens). I assume this is because of remote accessing - perhaps by those with personal motives if that is what the structure depends on - rather than tampering with the microscope when I was out or change in my own vision from one day to the next (I could see the greater opacity in the eyepiece lens yesterday, although that seemed not to be the problem today). On the other hand, I also had more problem taking the photograph, the time between my clicking the camera and the photo being taken varying and the photography more often being taken as my hand has shaken away from the object. Aware that the credibility of my argument may have been undermined by the small number of observations, particularly since acquiring the Apex Learner, I am now attempting to at least switch it on once a day and overall am pleased at the clarity of the object with the higher magnification lenses and at being to take any photographs at all. To clarify one thing that I observe when I adjust the focus, some objects present, notably in the case of the wrappings, the black edge and objects jutting from it, dissolve while other objects remain present at more or less the same degree of clarity, which is not so different from what is seen with magnifying lenses except that the distortions give an impression of structure and complexity.
(ix) I saw what looked like magnification of about 25 x with the 20 x ocular lens and 4 x objective, but may be overestimating the width of the screen; I didn’t estimate for the 10 and 40 x because of the brightness of the screen in the evening but took some interesting photos at each of the magnifications with the 20 x lens. With the last couple of observations, the distinction between light spots and objects seems to have ‘blurred’ since they disappear less quickly and accompanied by more changes to surrounding areas, but this may have been to do with the positioning of the piece of wrapper and more observations will be needed to establish the extent to which apparently complexity is ‘light spots’ as well as irregularities or distinctions in the film or particles on it.
(x) Since the last observations in December I have acquired a Celestron 600x optical microscope. So far I have only done observations using the mirror and external lighting (kitchen spotlights). I have been able to see clearly only at stated magnification of 80x, although the image at 80x is considerably darker than at 40x. At higher magnifications I can see nothing clearly outside the microscope. I can see, for example, the red rim around the sample (feather) on one of the slides at the same shade as it appears on the slide but it is not clear what part of the rim I am seeing or that I am seeing magnification. The fact that I see a circle instead of part of the rim suggests some sort of inversion of the image, and, in fact, at lower images I do not view the image directly: for instance, the labelling that appears upside down on the lower right of the slide appears instead on the upper left of the image, the right way up. The magnifications are significantly lower than those claimed. During two observations (23.2.18 and 2.3.18) I did not estimate consistently, guessing magnification of about 7-8 times on the first observation at between 40 and 80 times, and 10x and 25x for 40 and 80x stated on the second occasion (with the size of the screen estimated the second time to be around 40mm with the 10x ocular lens and around 70mm with the 20x ocular lens, although I will need to re-estimate the next time by comparing with dawn circles rather than by guessing against the tape measure. At 40x to 80x the complexity I saw on the feather slide did not appear significantly greater than under bright light with the eye alone (I need to examine the feather and onion prepared slides under a bright light also). I will do another observation without batteries alone to check for consistency and with the wrappings I used for the Apex Learner and Sunagor MagnaScope observations before repeating observations with batteries.
(xi) This time (6.3.18) I observed the lens wrapper under the Celestron again using the mirror only. At 40-80 I was able to observe the wrapper clearly, and also observed the increased size of the screen and focus on a smaller area, consistent with higher magnification as I adjusted the ocular lens between 10 and 20 x. Although there was complexity, the image did not seem to resemble what I’d seen with the Apex Learner and this was confirmed by my once again looking at the wrapper under the Apex, with the lower light since there is no mirror with the Apex. When I then returned to view the wrapper under the Celestron I had the illusion that the image had changed to resemble that of the Apex Learner. Unfortunately I didn’t look for long enough, deciding instead to view it under the light I had looked under it before in another room. By then I saw the initial image, as I did when I returned to the second light, and so the idea that the image I was viewing under the Celestron was being manipulated externally seemed less likely to have been ‘illusory’. In fact, the image I obtain with the Celestron resembles that I see with the top light with the Apex, the difference between the two images at the same magnification implying the importance of light in terms of the content of the image. I was unable to view the wrapper at higher magnifications with the Celestron but will repeat the observation once I have acquired batteries.
(xii) Repeating the observation under natural light (daylight) form the mirror, I was able to observe the image at the higher stated magnifications, ie, with 150 and 300, but only vaguely, not because it was blurred but because of inadequate lighting. With the higher magnifications I noticed a smaller final screen as the initial screen increased in size, which would seem to support the idea that a smaller area is being observed and reflected. I will re-observe with batteries. Again the image I saw resembled that obtained with the top light of the Apex Learner, a ‘negative’ image of that obtained with the lower light, so that the part of the screen with no object on it appears black, I assume because of reflection of the external mirror in the case of the Celestron and perhaps indicating reflection of an internal mirror in the case of lighting from above with the Apex (it looks like it will be difficult to take the Celestron apart). Shades of dark were more obvious this time in the image of the wrapper, so I am more likely to think I was wrong to believe that when I viewed the image under the Celestron immediately after viewing the same object under the Apex that I was viewing in each case a remotely constructed image.
(xiii) I then (7.3.18) repeated the observation under both daylight and with battery lighting, although only using the upper light, not having realised I could light from below by tilting the mirror. With battery lighting at 40x to 80 x the image was more or less the same, although there were light patches in some areas and some areas could be seen somewhat more clearly than with only daylight. I was able to see a larger screen, around 100 mm, at 300x to 600x with natural lighting, although the image was elusive, and I stopped the observation before trying to view with the battery.
(xiv) Reflecting on the last observations, I was reminded that the difficulty of finding the object on the slide as one increased magnification in the sense that one had to manipulate the slide more was consistent with focus on a smaller area and therefore reflection rather than refraction. On 10.3.18 I did another observation with the Celestron, this time with lower lighting with a 2mm wide strip of film that I had previously used with the Apex Learner. With the lower light the image initially seemed similar to that with the upper mirror until I adjusted the mirror, when the ‘negative’ effect receded, ie, light areas became less dark. I noticed that when using natural light to view the image I was able to see a vague image of my finger - a pinkish wash - when I put it against the end of the 30 x objective lens tube, I assume implying light entering the microscope from one of the other objective lens tubes, unless the eye itself were illuminating. I also had the fleeting impression that as I moved the slide it was the image above that of the wrapper - ie, the ‘floater’ type image that I assume to be the lens itself - that moved rather than what was below it, but this impression was not retained. I had difficulty seeing a clear image of the wrapper with the 15x and 30x objectives, and of finding a larger screen rather than ones of 10mm diameter or so, but then as I went back to the 4x had an impression of viewing a prepared ‘slide’ as opposed to a direct or even a new reflected image. However, I was able to then see the film at higher magnifications when I had removed it from the slide and, as with the Apex Learner, placed it directly on the stage, although this seemed surprising given that the lens tube was not then close to touching the object. However, the image at 10x ocular and 15 x and 30x objective lens tube was fairly clear, although with a small screen and I took some time to find it after seeing it initially as I swapped from 15x to 30x and then lost the image. There was a ‘fringe’ type effect at the edges of the small screen, as though one were seeing something resembling an eye lens, although I took this to be a ‘red herring’. Although my initial impression was of a similar image to that obtained with the 40x to 80x, there were significant areas of light, but as with later observations with the Apex Learner the image blurred rather than the light areas disappeared as I altered the focus. In fact, I had looked first at 40x to 80x and then 300 x to 600x and my impression was that as I then went to 150 x to 300x I was seeing a more opaque image with wider strips of black than with the 300x to 600x although this might have been to do with an area of black appearing as a single object rather than a number of objects rather than an operative not having realised the order in which I had viewed the object under the lens. Although the size of the screen would make estimation of magnification difficult at higher magnifications where the screen needs to be manipulated (I appeared to obtain a magnification of about 30x to 35x at stated 60x - ie, with the ocular turned about half way between 10x and 20x), I can still estimate magnification by focusing on objects within the screen (as I did sometimes with observations using the Apex Learner), including a tape measure at lower magnifications. Initially I had not realised that the microscope could be lit from the bottom. While variation between the image I obtain of the wrapping with the Apex Learner and Celestron using the lower light can be explained in terms of different illumination (although this in itself raises questions about the accuracy of diagnosis) and I cannot light the Apex Learner without the battery, in my next observation I will try to compare the image obtained with the MagnaScope and Celestron at similar low magnification and to repeat the comparison between the Apex Learner and Celestron using the lower light.
(xv) Interestingly I found a fairly clear and detailed image of what was beyond the microscope for the first time with the Prinz 2801 although did not carry out this observation for long because of hte lighting conditions - a fairly white light onto the mirror. The image appeared to be upside down as well as horizontally back to front. I suppose the earlier difficulty was a combination of my having viewed stained objects and perhaps stubbornness to attempt to see beyond the microscope once I had decided I could not, although it is possible I was seeing a constructed image; this might be supported by the fact that although I was seeing a variety of complex images according to which part of the slide I was viewing, I had trouble initially seeing anything when I changed to viewing a piece of translucent plastic bag with writing on it (to estimate size) and a piece of cotton thread. The magnification I initially saw with the thread then seemed to be blurring but because of the light I stopped the observation. With both the Celestron and the Apex Learner, and I cannot now remember which I looked at first although think it was the Celestron because I was interrupted at the door, I saw a far more detailed image of the frosted glass on the slide than I had noticed previously, so that I had some trouble distinguishing the slide from the object; the chocolate colour of the image did have a constructed look to it. With the Prinz the image had been lighter, with a ‘rippled’ look, as though one were viewing a powerpoint transition effect. I will repeat the observation with the same slide and next time all four microscopes when there is less obvious glare.
(xvi) Repeating the observation with the Prinz 2801, I was very surprised to see an image of the lens wrapper very similar to that I had with the Apex Learner at 40x, that is, a complex image without light spots, with a magnification, based on an object on the screen of 25 x plus with the 4x objective lens and 15x ocular lens. However, I cannot rule out that the presence of a complex enlarged image beyond the microscope for the first time is not due to my having become more adept at using the microscope, since I have only just noticed also that the apparent focal adjustment is actually the mechanism for changing ocular magnification from 10 to 15 x, whereas the focal adjustment is, as with the Celestron, at the sides. So it may be that I simply never had an object in focus! This does not, however, invalidate my hypothesis, so that I am either overestimating magnification - which cannot be measured, only estimated - or that the object is detected and a larger image constructed.
(xvii) What seemed initially like an exciting observation with the Sunagor MagnaScope was less so when I went back the next day to look at the object: I had thought a ‘hair’ had been incorrectly detected on some bird dropping on my garden table, that it was instead the grain of the table next to the dropping, but the next day when I went back I found the hair. I noticed that with the Sunagor I am viewing everything the correct way round - ie, if I move the object to the right, the image also moves to the right. I had been lucky enough to photograph the object with and without the microscope before the batteries exhausted. April 2018.
(xviii) I repeated an earlier observation with the onion epidermis prepared slide that came with the Celestron. I was able to see an attractive turquoise image with all four microscopes, divided into ‘tiles’ or rectangles visible in each of them. Darker blocks at one end of the piece of epidermis were just visible to the eye if one held the slide up to the light and were visible as darker blocks when viewed under the Prinz and Apex Learner but not, surprisingly with the Celestron and Sunagor MagnaScope. With the Prinz, I was able to see the object clearly at 40x only. According to my observation, I was able to obtain an image up to 600 x (despite not usually being able to focus at 600x because of the thickness of the slide). At 600x stated I estimated magnification of about 100x based on the diameter of the screen and the speck being 1mm (although it may have been less). Although the magnified image looked unremarkable but realistic, it is surprising that the darker shape - assuming it is a darker version of a regular shape rather than a speck of some sort - would magnify so clearly at 100x (10,000 x if one thinks of height x width). Apart from the tiling effect, there was no other complexity. With the Celestron, the contrast between the turquoise and white was more striking. If I squinted I could just see a lighter line down the middle, as when I held the slide to the light, even if I couldn’t see the darker tiles on each side - otherwise I might have thought I was looking at an image prepared for a different slide. In fact, however, when I went back to the Apex Learner, according to my notes, the image was not consistent with the object in that the side that had more dark cells was one that had different lettering (the label of the slide) near it. This was an interesting observation, whether or not I would see it if repeated in that I was certain at the time of the inconsistency. I also observed that while the lettering is the wrong way around with the Apex Learner (ie, as though viewing it in a mirror), and the image is rotated with the Celestron, with the Sunagor it is the right way around and not rotated.
(xix) I began an observation with a bit of snail tissue and then unfortunately lost the sample. The sample looked a striking black shape at 40x and 100 x lit from below with the Apex Learner. Although I obtained a complex structure with the top light, the structures did not in any sense, at a magnification of 40x, resemble anything like a cell. I was able to detect the shape again at 100 x before losing the object, first when I tried to view it with the 40x objective lens so that it stuck to the lens as I adjusted the focus and then when I lost a smaller part of the object from the slide. I did not attempt to photograph the object and image before losing it. April 2018.
(xx) I began to look a piece of my fingernail under the Apex Learner microscope, cut to 1-2 mm, and found the image resembled that of a piece of wrapper - more Jaffa cake than lens wrapper - at 40x and 100x. The images was of a pattern of dark and light with many small parts, a fairly uniform pattern, with the small parts being difficult to pick out at either 40x or 100 x but looking something like parts of a circle or corners of a square. At 400x I was not initially able to find the object despite removing the nail from the slide and holding it under the lens,but was able to see it fairly clearly the next time I tried, but not then subsequently. Also, initially I thought I was obtaining the same image - and subsequently the ridges on the nail became more evident - whichever side of the nail I looked at but subsequently had more trouble detecting the image when the shiny side was nearest to the lens. The light from the Apexi Learner then seemed too bright (unlike with the Prinz when too much light from an external light source will blur the image, the screen on the Apex Learner can seem very bright with the image still clear) and so I will repeat the observation with the Celestron under brighter natural lighting conditions and also to find out what the size of a cell in a finger nail might be,a lthough I assume it would be too small to view even at stated 800x. April 2018.
Conclusions about the diagnostic ability of microscopes from observations of magnifying glasses and optical microscopes.
As well as the observed magnifications differing from those claimed by the manufacturer, there is is also variance between the magnifications said to be needed to view the cell and the apparent size of the cell. The HIV viral cell is said to be one time ten to the minus nine millimetres in diameter (NIH, 2013) and a magnification of 10,000 is said to be needed to view the HIV cell (NIH, 2013). The image of the cell would then be 0.01 millimetre in diameter – ie, too small to be able to view the cell. (This also assumes that magnification of 10,000 x is magnification of diameter rather than area – if the latter, then the image would be proportionately smaller, and even the highest magnifications apparently capable of being achieved with the electron microscope would not be enough to view cells and diagnose disease.)
“Straight wave fronts change direction when they travel at an angle across a boundary between deep and shallow water. The waves have been refracted at the boundary because the wave speed in deep water is greater than in shallow water.” In Jim Breithaupt, Understand Physics for Advanced Level.
Before finding apparently high magnification with the Apex Learner, I had sought to establish the limits of magnification by looking at magnifying lenses. Unfortunately, although I have acquired magnifying lenses, I did not think to label each of them after taking them out of their packaging but have estimated actual magnification. The magnifications are surprisingly low in the sense that one has the effect of higher magnification than there actually is if magnification is considered in terms of length only. Of the 12 lenses I have ,most of them magnify clearly only at about 1.5 x, with the highest clear magnification appearing to be three, although it turned out to be less when I compounded it with another lens, when the total magnification was still three. Distortions included tunnelling, accompanied by a kaleidoscopic effect, elongated shadows of objects away from the centre of the lens, and multiple images, as we lift the lens from the object or our eyes from the lens and focused more on the lens itself. With the lens from the Sunagor MagnaScope I became aware of the extent of magnification achieved by projection to the lens to the eye (with the magnifying lenses there tended to be tunnelling rather than much of an increase in size), so that by standing above the lens I was able to observe relatively clear magnification - ie, enough to easily recognise a letter - by as much as 20 (more than with the microscope, ie, with the zoom attached, where I have only seen 15 x, based on a screen of around 100 mm). I also noticed the image blurring and then turning upside down as I lifted the lens and funnel from the page. However, what I also noticed was that instead of direct viewing we are seeing a reflected image, as well as that distortions, blurring and loss of visibility occur fairly quickly.
First of all, It is difficult to see how a lens would ‘stretch ‘an object so we view it in the same position it is located, especially when a convex lens focuses on a smaller rather than larger area. Within the lens itself refraction may take place but the projection of light will be impeded rather than enhanced because there is greater resistance so that the angle of incidence is less. If the principle were refraction, this would depend, as is hinted at in the quote above, on the ‘speed of light’ within the heavier material being faster rather than slower and offering less resistance and therefore reducing rather than increasing the angle at which light entered the lens (unless it were perpendicular, in which case one would expect the angle to remain the same), which seems unlikely. In fact, when a lens is placed in front of a slide projector or a torch behind a lens the image becomes smaller and there are distortions. Also, for significant refraction we would also expect the whole of the area of the glass circle on the microscope stage to be illuminated rather than a part of it, which supports convergence from the lens rather than divergence within it; instead, as we increase magnification, we may need to move the slide around before find an image and can sometimes see a smaller area illuminated. On the other hand convexity cannot alone explain enlargement even if it might lead us to focus on a smaller area and therefore perhaps, especially with better illumination, see with somewhat more clarity. []
Reflection can explain enlargement and it is also what we see if we observe closely. With the correct lighting a magnifying glass will see objects behind us more clearly than those in front, which can only be accounted for by reflection. Reflection also explains why the image turns upside down – we see it do so – as we move our eyes away from a magnifying glass, when we begin to see the front of the lens rather than the back of it, therefore implying that convergence and divergence occur within the lens (although this requires further thought and elaboration). It is also ‘on reflection’, what we see: we appear to view directly when a magnifying glass is on the page but almost immediately as we lift it the image looks more like a reflected one. Reflection allows, in theory, for significant enlargement: the angle of reflection can, as with light projection, easily be higher than the usual stated angles of reflection and the object may be reflected onto one face , or lens, and then may be partially re-reflected onto the other so that only a part of the image appears but at greater magnification.
Enlargement is then explained by the ratio of reflection from the object to the lens or lenses, determined by the shape of the lens and its diameter and a converging or diverging light source, and the relative positions of the object and lens. Reflection occurs onto the surface of the lens as though it were being projected from the image, whether or not the angle of enlargement is also dependent upon the angle of additional illumination. Reflection then occurs within the lens - additional enlargement depending not on refraction but on partial re-reflection for a given angle of convergence and divergence so that only a part of the image appears within the lens, the part we observe. As we lift a magnifying glass, a smaller area is reflected – even if we can have the illusion that divergence is taking place – so that individual objects appear larger. If we move our eyes away from the magnifying glass, the image also increases, or appears to, as divergence from the lens appears to continue. As we then continue to lift the glass, it focuses on a larger area as the influence of convexity recedes or reverses so that enlargement is reduced but may still be achieved to an extent by our focusing on a smaller area than with the eye alone because of the size of the lens. Reduction will also occur as we instead focus on the front side of the lens if the front side of the lens is also convex.
The apparent paradox, in terms of reflection, whereby as we appear to focus on a larger area as we raise a lens from a page the individual objects on it also get bigger can be explained by the fact that although we think we focus on a larger area as we lift the lens, there is in fact convergence, not divergence, as we would expect from a convex lens, so that individual objects appear larger because fewer of them are being reflected. The reason we see the image the right way around is that we are viewing the back of a reflected image – ie, as though we are looking at the back of a translucent mirror. Rather than there then being divergence from the concave face of the lens it seems likely – at least from the fact that when we look at an object placed in a glass of water it looks a similar size to when we place it beyond the glass – that there is also convergence from the concave face of the lens.
According to El-Kareh and El-Kareh, in 1970, magnification with the electron microscope is said to be accounted for by refraction and phase differences. While the latter will be discussed in a later section (it is not included in the book’s index), the inadequacy of refraction as a mechanism or principle to explain enlargement seems to be referred to fairly explicitly. Beams of electrons are said to be refracted in the same direction as ordinary light and the potential distortion is hinted at in this quote:
“The refractive index for light is usually contained between the limits n + 1 and 2.5. In electron optics, the range of refractive indices is enormous. Values as high as 1000 are often used. … A substantial proportion of an electron beam passing through matter will be scattered incoherently with resulting energy loss to the specimen.” A.B. El-Kareh & J.C.J. El-Kareh, Electron Beams, Lenses, and Optics, Vol 1, 1970.
Reflection allows, in theory, for significant enlargement: the object may be reflected onto the face, or lens, and then may be partially re-reflected within the lens or onto the other if it is concave so that only a part of the image appears but at greater magnification. On the other hand, whereas with slide projection the factor in enlargement is the distance from the diverging light source and the strength of the light, with lens enlargement, the lens or light source might seem to have to be of an unattainable precision if a very small area is to be illuminated so that a significant area is reflected, especially if the lenses onto which they are projected are also small, as is the case with the lenses in the objective lens tube and the eyepiece. The fact that we do appear to obtain very high magnification with, for instance, the Apex Learner despite the size of the lens tubes lends further support to the idea that the lenses are not responsible for the size of the image. []
A point of interest about reflection is that there may be reflection from the lens of the eye itself as well as from the microscope lens, whereby it acts as a mirror and light source. For example, if we lift our eye from the magnifying lens of the Sunagor held in front of us we see objects beyond the lens on the periphery of the lens, whereas we see a blur if we have our eye to the eyepiece. When I looked into the glass square cut near the top of the otherwise opaque metal box in the science room in Newark Museum, in Newark, New Jersey, in 2016, I saw objects beyond the box reflected in the glass, which again implies that objects were reflected from my eye onto the glass. That this suggests reflection in the lens of the eye may be supported by observation that the image of an object becomes smaller if we block part of our eye, as though the image were diverging from a smaller mirror. Also, this would explain why the objects in an otherwise two-dimensional mirror change as we view them from a different angle, their being reflected in the mirror at the angle at which we look into the mirror.
Whether or not magnification occurs as a result of reflection, with magnifying lenses we notice blurring and distortions at low magnification and should therefore be sceptical that the clear image we can apparently see at very high magnification is an enlarged one. As we increase magnification when using a lens by lifting the lens, we notice blurring and sometimes colouring at the edges. One possible explanation is restriction of partial re-reflection by the rim or cylinder, the effect increasing as less of the image is reflected: some restriction or limitation of partial re-reflection would cause blurring at the edges of the image and eventually causes blurring or loss of visibility of the whole image as the edges are forced back onto the lens. On the other hand, partial re-reflection must account for the magnifying effect of convex lenses or they would presumably be two dimensional. The un-re-reflected part of the initial image may in fact be more likely to escape than remain within the cylinder or be reflected back although the cylinder might account for blurring or colouring the edges. Another explanation is that we are seeing an increasingly weaker image as a result of re-reflection and this is most apparent at the edge of the image, where the image is most ‘stretched’, the bronze colour we sometimes see being a result of weakening of the black within the glass of a black and white image. Or the blurring and colouring may be the result of over illumination as the glass is lifted, in the same way as colour distortions occur mainly with the stronger of the two lights on the Apex Learner. There is also a tunneling effect with thicker or multiple lenses, where successive images become apparent. One explanation is that for a given size and thickness of lens there is initial reflection onto less than the whole diameter of the lens and then re-reflection onto more of the glass; the succession of images also explains blurring and colouring at the edges. Blurring is also the result of an increase in size, as lines become less defined, as well as over illumination, while loss of visibility may be the result of over illumination or, in theory, although this needs further thought, divergence of light at 90 degrees from the lens so that enlargement is theoretically infinite.
Nor is the microscope necessarily the optimal system for obtaining - or viewing - an enlarged image. When we look into the cylinder we see a brightly illuminated screen at the end of it at what appears to be about the position of the condenser or beyond it. The cylinder itself appears dimly lit, which need not prevent compound magnification, as we can tell by using a zoom lens (assuming the lens is in the correct position relative to the eye) even given the small size of the apertures, and even though, unlike with a zoom lens, if you hold the microscope upside down and look into it you cannot see far inside. Nor need the light to the slide necessarily be encased from the final lens to the slide, as again one can see from using a zoom lens, although given the kind of precision we would need to magnify a speck to the width of the lens, we might expect this: the Sunagor MagnaScope has a funnel that enables clear viewing of enlarged objects but objects beyond the funnel appear blurred because of diffusion (the light is not strong enough or the ratio of reflection approaches infinity).
With the standard microscope the slide is placed at the normal distance from the eye, which suggests the microscope is not constructed to optimise magnification of what is on the slide.
If there is a lens at the top of the microscope, we would expect it to be further from the eye to maximise magnification: instead we place our eye over the eyepiece or very close to it so that we can see inside the aperture through the small cylinder. Similarly, we might expect the slide to be further from the final lens, although in fact the size of the lens and apertures is such that in fact the slide ought to be even nearer. Although the condenser is sometimes described as a lens, we would also have difficulty viewing it directly: instead we would be more likely to view a screen in the cylinder or cylinder and rack. The fact that the microscope views something very close and the telescope something very far away might seem intuitively not to make sense, although less so if we think of the telescope as similar to a zoom and the microscope to a magnifying glass: the telescope is a long way from the object because it has to be. However, if we look inside the telescope, which I did by chance because the top came off, we see that it contains only mirrors, one at the base of the cylinder and a smaller one near the top, placed at 45 degrees. If it does not prove it, this at least supports the idea that one of the processes taking place in enlargement is reflection.
If the microscope were to increase the size of the object whether as a result of direct viewing or reflection within the lens and cylinder of the microscope, the increase in size would be at the expense of clarity, whether or not this is inherent in enlargement, because of the thickness of the lens, compounding, the darkness of the tube, or distortion caused by the brightness of the illumination. With higher magnifications with lenses we see a blur, and this might even be intended to be a feature of the the construction of the microscope: for example, the aperture may be positioned in order that a constructed image or internal object is viewed more clearly. Why, then, for example, the structure revealed with the strongest lenses with the Apex Learner can be seen when manipulated by hand without a slide present is not clear, although this example does at least support the idea that construction is not always accurate or optimal.
Given that enlargement is determined by the ratio of the object to the lens face, we would expect an optimal system to use larger lenses to compensate for limitations in the precision of convexity and illumination: instead the objective lens tube in the Prinz 2801 microscope measures only 10 mm and the condenser, including the rack, at most 50 mm. A smaller diameter also would not overcome, but increase, the distorting effects of thicker and/or more convex lenses. A very small lens might increase enlargement, depending on the illumination, when the point is to project an image of an object contained within the microscope but not if the point is to view or reflect something beyond it clearly and at high magnification. As well as the ocular lens being larger than the objective lens, the objective lens is larger than the aperture; if we expected direct viewing of an enlarged object, we would also expect the aperture to be larger than the lens. Because the ocular lens is larger in diameter than the objective lenses, then assuming a similar convexity, we might expect a reduced, not enlarged, image of the object beyond the lenses because if we turn a a zoom such as the one of the Sunagor MagnaScope around the final image appears smaller but, I think, because a given length of the zoom, or microscope cylinder, produces a smaller virtual image in the second lens rather than because of the respective sizes alone (from observation of lenses). In any case, the ratio of the width of the lenses to the material on the slide or the diameter of the cylinder is smaller than one would expect to optimise significant enlargement of the object, whether or not there is partial re-reflection within the lens.
In the Prinz, the apertures at the end of the objective lens tubes are also smaller than one would expect, given the size and height of the lens tube and the enlargement claimed. This means either of two things: First, if reflection is determined by light projection from the mirror, then there will be crowding at the entrance to the aperture because the angle is too large and the object will be reflected onto only a part of the lens, so that, given the constriction of the lens tube, enlargement is not maximised. We would also expect blurring as a result of diffraction, or the crowding of light around an exit, which undermines the function of the condenser. First, if reflection is determined by light projection from the mirror, then there will be crowding at the entrance to the aperture because the angle is too large and the object will be reflected onto only a part of the lens, so that, given the constriction of the lens tube, enlargement is not maximised. We would also expect blurring as a result of diffraction, or the crowding of light around an exit, which undermines the function of the condenser. Second, if reflection is determined by convexity then if the angle of reflection is such that it passes through the aperture at the correct width, then, as the calculation shows, there is convergence before the slide so that we would expect to see a blur or at least a less than clear image. On the other hand, the smaller aperture, and lens, may act as a condenser in that a larger area is reflected before being magnified by the convex lens.
With the standard microscope (the design of the Prinz 2801), the size of the screen appears to be about the same as the size of the condenser, which is to say about 8 times the diameter of the objective lens tube, rather than the magnification of 10 or 15 x we would expect if the ocular lens were compounding magnification. However, given the construction of the microscope, we would not be able to view the condenser directly so that it is more likely that we are viewing a smaller – 6x - screen or lens within the cylinder tube and adjoining rack. Also, with varying magnifications in the three objective lens tubes we might expect lens tubes of different diameters and/or different lengths. If not, then we would expect the lens with the highest magnification to have a smaller, not larger, aperture because we would expect a greater angle from the lens: in fact, the aperture for 60 x is larger, not smaller, than that for 30 x, which would imply that the lens for 30 x would need to be proportionately smaller in diameter.
Before I had found apparently high magnification with the Apex Learner and after direct viewing but at significantly lower than claimed magnification with the Sunagor MagnaScope, I believed I was correct in thinking that the largely unchanging image one saw with the Prinz did not mean that the microscope was faulty but that the more complex and moving structures one saw with a microscope were of something within the microscope itself. With the Prinz, I did not appear to be viewing what was beyond the microscope with a standard microscope, apart from the stain, which appears as a pale, undifferentiated ‘wash’ over a fixed image on the screen. Instead we see something resembling in structure the lens of our own eye, as it appears when we squint looking into sunlight, on a relatively fixed screen. We may also in fact see the lens of our own eye in layers above the screen (see above), as we can also with the Sunagor and Apex Learner. And if we turn the Prinz microscope upside down, we see what looks like the iris of a small animal or bird.
If we rotate the focal adjustment of the Prinz, the image rotates with the cylinder wall, which implies we are viewing an object inside the cylinder. However, we see a similar small circular image if we hold the magnifying glass of the Sunagor above a glass bowl. This supports the idea that we may see the lens of our own eye as well as the object contained within the traditional microscope. On the other hand, what we may be viewing, if there is no object present, is the lens itself reflected at relatively low magnification. The stain on the slide appears as a pale ‘wash’ over the fixed image. In any case, what we are not viewing, except at much lower magnification than is needed to view a ‘cell’ and of an object that is not translucent, is the sample on the slide. The higher observations with the Apex Learner did not make me doubt that microscopes do not diagnose disease - the point of studying the microscope was to prove something I knew to be true rather than to found out whether or not it was true - but did lead me re-assess the light microscope and what it could achieve until I realised that all microscopes were electron microscopes in the sense that light and lenses alone do not produce the complexity visible on the screen.
“The electron microscope has not yet been very useful in the study of the chromosomes, and most of the information about their behaviour during cell division comes from light microscopy and, latterly, from biochemistry.” R.J.C. Harris, Cancer, 1962.
It is difficult to find out when the electron microscope was first invented: encyclopaedia entries conspicuously leave the electron microscope out of timelines on the history of electronics, although I may have remembered, but cannot attribute, seeing a reference to 1948. Harris, in 1962, writes as though the electron microscope is a fairly recent invention, and in 1970 El-Kareh say that the history of electronic optics is only 30 years old. Dorland’s medical dictionary, published in 1900 but my edition 1957, lists the electron microscope but not as the microscope with the greatest magnifying power: these are instead the corneal and ultramicroscopes. Hutchinson says that the most modern electron microscope can magnify up to “7 million times (7,000,000 x)”, the ‘x’ as well as perhaps implying one can substitute any high number one wants also reminds us that enlargement is usually associated with area, even though the zoom lens is measured in distance; Dorland, however, only mentions 100 ‘diameters’ for the x-ray microscope but not for the other types.
The principles of reflection and projection remain the same whatever the actual or stated variation in the method of illumination and enlargement. Materials of different quality may have some impact, in terms of opacity and distortion (or resistance) but will not have such a significant impact that higher quality, or newer, materials will allow magnification by hundreds of thousands of times. Resolution – ie, the clarity of an enlarged image – depends on the opacity, size, convexity and position of the lens: unless an image is reconstructed electronically, the principle remains the same as in the standard microscope.
An electron microscope is said to work using electron beams instead of a conventional light source, with magnets taking the place and fulfilling the function of lenses. The reason electron beams are used is because traditional light sources are said to have wave lengths that are too long for the high resolution needed to see the cell. It is said to allow viewing of, for instance, the influenza B , polio and herpes virus at a magnification of 100,000 “x” (National Institute of Health, (www.ncbi.nih.gov/pmc/articles/PMC2772359). Whereas with the eye alone, we can apparently only discriminate between objects one quarter of a millimetre apart, the electron microscope can identify objects 1,000 times closer together than this and view objects clearly at a magnification of up to 7 million times.[reference needed]
Setting aside the question of how electrons are isolated , converted into beams, or how stains can be made electron dense, or why they illuminate in a way that is any different from ordinary light produced by a battery or electric circuit, the principle would seem to be asking too much of illumination, in that better lighting might produce a clearer image at the same size, as would a less opaque medium through which light has to travel, but will not ‘resolve’ the problem of how a clear image can be obtained of an object at very high magnification: we are not seeing an object more clearly, unless we are bringing an object closer or compensating for any fault in our own vision. Second, in what way could electron beams magnetic coil enlarge such that this substantially increases magnification? The diffraction, or scattering, from (SEM) or through (TEM) the image might – hypothetically - be more precise than convergence from lenses, but whether or not this is a plausible process, it is not clear why the image itself would be larger or more precise at higher magnification than that achieved by ordinary projection. Phase difference, the second principle, in addition to diffraction, appears to be no more than electrons responding to more or less translucent or opaque areas of the sample in the same way as light would. [Third, in what sense can a stain on the slide be ‘electron dense’ – such that it aids illumination more than the glass beneath it?] Images that appear to show underlying structure are constructions. Reconstructions using, for example, pixels, aside from the fact that they are reconstructions, offer higher ‘resolution’ because the smaller the part the less coarse the image, but that is an illustration of the fact that enlargement weakens the image in the sense of making it less clear.
In fact, the term ‘electron microscope’ might be implying that all microscopes nowadays are electron microscopes in the sense that they might be – electronically – viewed and manipulated externally. This is supported by the stillness and photographic quality of the image of the bronze wrapping viewed under the Apex Learner. Although this will need further observation and reflection and some reading about electronics and magnetics, I now assume the enlarged image is one detected by a magnet of some kind in the condenser or elsewhere in the microscope , whether after or without initial reflection, which is then enlarged and given a complex interior by operatives external to the microscope, whether or not in real time, to account for variation and determine the outcome.
Appendix 1: Photographs taken during observations
Figure 1
Bronze lens wrapper film 200 x stated. Early November 2017.
Figure 2
Bronze lens wrapper film 80 x stated. Early November 2017.
Figure 3
Orange Jaffacake film wrapping 800 x stated . Early November 2017.
Figure 4
Lens wrapper 800x stated (taken after the previous photo and with less clarity of image and more difficult positioning of the camera). Actual magnification approximately 300 - 400 x (the piece of film being about 1-1.5 mm, although closer to 1 mm, and moved across approximately 4 screens), less than during my initial observations but consistent with later but also clearer previous observations.
Manipulation of the piece of film was also more difficult than during earlier attempts, mainly because the whole of the screen was no longer in focus.
(20.11.17)
Figure 5
The same piece of film, also at 800 x stated, taken from a different position. (20.11.17)
Figure 6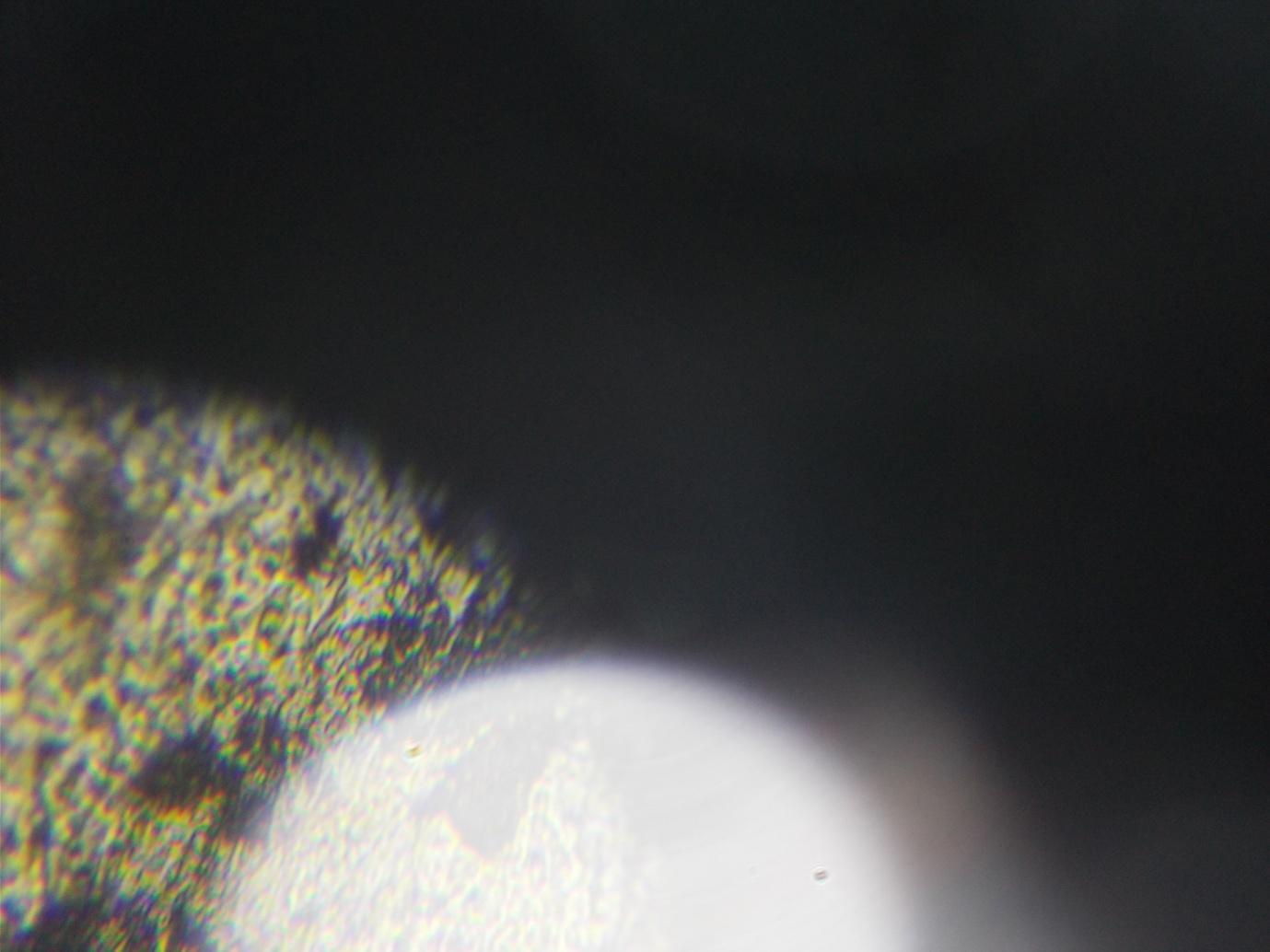
Lens wrapper. Stated 200 x. Actual c 130 x. (20.22.17)
Figure 7
Lens wrapper. Stated 80 x. Actual c 75 x.
Figure 8
I think this is also at stated 800 [see observations]. This was also taken earlier in November 2017 with less clarity than initial observations. Initially both the black line and the object below it disappeared as the focus was adjusted, appearing to be light distortion, and leaving the rest of the image relatively clear. With subsequent observations, both the light distortion and the rest of the image blurred as the focus was adjusted.
Figure 9
I think this was at the same magnification with the camera closer to the eyepiece lens, which would be the case if the last label is correct.
Figure 10
[check observations] The same piece of Jaffacake wrapping (the top of the width of the film) at 200 x stated.
Figure 11
The same piece of wrapping at 80x stated.
Figure 12
80 x stated piece of film lit from below.
Figure 13
At 80x stated, a piece of film wrapping lit from below.
Figure 14
This was the top of a piece of light distortion sticking out from the edge of a piece of film wrapping, from memory and the size of the distortion, at 800 x stated.
Figure 15
This photo taken into the cylinder of the Sunagor MagnaScope shows what looks like a hair on the right of the photo at the edge of the unseen circle. When in the centre of the circle of bird droppings, before I started to prod it to see if it was a hair or not it looked more obviously like a hair and slightly above the object, although I think one can see from this photo that it is not obviously part of the table it is resting on.
Figure 16
Here is the photo of the circle of bird droppings. The ‘hair’ in the last photo is at about 11 o’clock, to the left of the small circle adjoining the the larger circle, although the first, not the second, line. When I took the two photos I was convinced that what I was photographing was not a hair but simply a mark on the object and a microscope operative had got it wrong, but going back today I see that unless the same bird decided to place a hair there I was wrong - it was a small hair on the edge of the circle, although I’m surprised I could see it yesterday, perhaps because I was looking at the mark to the left of the hair.
Selected quotations
"According to Babeuf, Robespierre expected the population to be greatly reduced by the Terror, the war and the internal uprisings. He planned to achieve a redistribution of the land by the liquidation of the landowning class. Its members would be, if not killed off, forced to 'execute themselves' in time, and in their own interest." J.L. Talmon, The Origins of Totalitarian Democracy, 1952.
"If radical environmentalists were to invent a disease to bring human population back to sanity, it would probably be something like AIDS ... the possible benefits of this to the environment are staggering ... just as the Plague contributed to the demise of feudalism, AIDS has the potential to end industrialism." Christopher Manes, Green Rage: Radical Environmentalism and the Unmaking of Civilization, 1991.
“The world revealed by a microscope is not less wonderful than the world revealed by the telescope. … The wing of the fly is not only a thing of beauty, but is cleverly constructed on sound mechanical principles; the eye of a fly is not only beautifully made, but shows a knowledge of optics;”. 100 Peeps Through A Microscope, in ArthurMee (ed), The Children’s Encyclopedia, Volume 3.
"The case of sickle cell anemia and malaria is rather a special one, but beneficial evolutionary consequences of improved medical care may be quite common." John Maynard Smith, Eugenics and Utopia, 1965.
"It follows that as our ability to recognise heterozygotes increases, we could be led to sterilize almost the whole population on eugenic grounds, which is clearly absurd." John Maynard Smith, Eugenics and Utopia, 1965.
"Vide Ricardo's letter to Malthus of October 9, 1820: “No law can be laid down respecting quantity, but a tolerably correct one can be laid down respecting proportions. Every day I am more satisfied that the former is vain and delusive, and the latter only the true objects of the science.'" John Maynard Keynes, The General Theory of Employment, Interest and Money, 1936.
"We might conjecture that in the long run, if there is an upper bound on ability, we would eventually reach a society with the greatest equal liberty the members of which enjoy the greatest equal talent. But I shall not pursue this thought here." John Rawls, A Theory of Justice, 1972.
“An institution suitably located, constructed, organized, managed and personneled, to supply, scientifically, economically, efficiently and unhindered, all or any recognized part of the complex requirements for the prevention, diagnosis, and treatment of physical, mental, and the medical aspect of social ills; with functioning facilities for training new workers in the many special professional, technical and economic fields essential to the discharge of its proper functions; and with adequate contacts with physicians, other hospitals, medical schools and all accredited health agencies engaged in the better health program.” Quotation from the Council on Medical Education, entry under ‘hospital’ in Dorland’s Illustrated Medical Dictionary, 23rd Edition, 1962.
“Apparent magnification under the microscope.” Under ‘magnification’ in Dorland’s Illustrated Medical Dictionary, 1962.
“This sixth edition introduces widespread amendments, which reflect the ever-changing face of opthalmic practice. To keep abreast of changes in examination techniques, I have added an appendix of Multiple Choice Questions, as well as a short glossary, for both of which I am grateful to the help and patience of Dr Stuart Pinkerton, and which I hope will ease the passage of the hard-pressed student. Patrick Trevor Roper, 3 Park Square West, London NW1”. Patrick D. Trevor-Roper, Lecture Notes on Opthalmology, Sixth Edition, 1980.
“The great conquerors, from Alexander to Caesar, and from Caesar to Napoleon, influenced profoundly the lives of subsequent generations. But the total effect of this influence shrinks to insignificance, if compared to the entire transformation of human habits and human mentality produced by the long line of men of thought from Thales to the present day, men individually powerless, but ultimately the rulers of the world.” Alfred North Whitehead, Science and the Modern World, Lowell Lectures, 1925, 1967.
“This work [volume I, 827 pages] is intended for continuous reading, being designed as a course of study. In dealing with an enormous field of knowledge the editors have thought it best to omit consideration of subjects adequately treated elsewhere in accessible works. They have excluded from their survey, for example, the development of medicine, of architecture, and of certain other arts.” Charles Singer, E.J. Holmyard and A. R. Hall, A History of Technology, Volume I, 1958.
“A microscope using light will not magnify things clearly more than about 3,000 times, whilst an electron microscope can already magnify up to 300,000 times, and will probably do much better in future. … So far as I know, no photographs have yet been taken to show the detailed structure of human cells, but this will doubtless be done soon if it has not been done already.” J.B.S. Haldane, Science Advances, 1949.
“Executive order 8840, dated July 30, 1941, established the Office of Coordinator of Inter American Affairs within the Office for Emergency Management. The Coordinator took over the functions of the Office of Commercial and Cultural Relations Between the American Republics established by order of the Council of National Defense on August 16, 1940. The Office of the Coordinator of Inter American Affairs coordinates all activities of this country, designed to improve cultural and commercial relations among the nations of the Western Hemisphere. It operates both through governmental and private agencies in such fields as the arts and sciences, education, and travel, the radio, the press, and the cinema.” In US Government Monthly Catalog issued by the Superintendent of Documents, No 564, December 1941, page 1697. The significance of this may seem less obvious than that of some of the other quotes, but there are many suggestions that history has been re-written and whether or not this is an authentic record, the emphasis on ‘inter American affairs’, and lack of reference to Pearl Harbour, although troops in Washington on December 12th, as well as a reference elsewhere to optical instruments and a research paper, RPI, 1430, on ‘Reflection-Transmission relationships in sheet materials’ by Herbert F. Launer, 1941, suggests one avenue of research into the history of when and why disease was invented, although there are likely to be blind alleys.
“Two contrary laws seem to be wrestling with each other nowadays: the one, a law of blood and death, ever imagining new means of destruction and forcing nations to be constantly ready for the battlefield; the other, a law of peace, work and health, ever evolving new means of delivering man from the scourges which beset him. The one seeks violent conquests, the other the relief of humanity. The latter places one human life above any victory; while the former would sacrifice hundreds and thousands of lives …..” Louis Pasteur, 1888, quoted in Kenneth M. Smith, Beyond The Microscope, 1945.
“Now the war is over, I am beginning to start work again on these problems, though I cannot do much till University College is rebuilt. The Nazis have done such horrible things on the basis of their false theories of heredity that all work on this subject is inevitably suspect. But we can best counter these falsehoods by discovering the truth, and the truth is going to take the study of human, animal, and plant structure a whole stage beyond that which the microscope made possible.” J.B.S. Haldane, Science Advances, 1949.
Appendix 1: earlier observations with the Prinz, Sunagor MagnaScope and Apex Learner [draft report]
The first observations were from the Prinz 2801, made while assuming that what one saw when the microscope was illuminated from the mirror was all one could see with the microscope:
The lenses are the wrong way around: we would expect to see a smaller image where the first lens is larger than the smaller for the length of the tube whether or not the eye also leads us to expect a smaller image. With the Prinz 2801 microscope we do not appear to see anything beyond the microscope except as a pale ‘wash’ of the stain over a more complex image of something within the microscope, although at the moment I have no way of knowing if a different illumination would produce different findings. With the Sunagor MagnaScope and the Apex Learner we see beyond the microscope and magnification is compounded – ie, both lenses add to the size of the image - but the image we view is at significantly lower magnification than claimed, although there is less ambiguity as to what we are seeing with different objects using the Sunagor: at high magnification with the Apex Learner, as well as the blurred image of the stain we see a structure similar to that with the Prinz and likely to be of something within the microscope, although this conclusion requires corroboration. With none of the microscopes can we see clearly at more than 100 times, and perhaps less, the imprecision due to our not being able to determine precisely the width of the screen we are actually viewing nor to distinguish between light distortion and enlargement.
The Sunagor MagnaScope recently appeared to magnify a piece of film cut to 1 mm around 4-5 times with the microscope (ie, with the zoom added to the magnifying glass). Apparent clear magnification seems intuitively to be higher when we first look at the image but seems to be because we overestimate the size of the screen, since if we compare the actual size of individual letters to the size they appear to be on the screen, the magnification appears to be closer to four to five times. If one removes the funnel of the microscope away from the microscope, the image blurs to the point of invisibility very quickly – ie, within a few millimetres – which implies either that the limits of convexity have been reached or, given the shape of the funnel and the blurring rather than reduction, diffraction of light. Enlargement with the microscope - ie, with the magnifying glass and zoom - is considerably less – around one fortieth - than the 196 times (28x and 7x) claimed and less than would be needed to see a ‘cell’. Enlargement can be explained by partial re-reflection from the magnifying glass lens to the lower of the zoom lenses (see section on Reflection). The magnifying glass appears to magnify clearly at around 2 x . As I lift my eye from the magnifying glass, the image blurs and then turns upside down. The zoom also appears to magnify about 2x, although the effect is to produce an image of around the same size as the object, as if we were standing at the correct position to view it in focus. One interesting observation with the Sunagor MagnaScope is that when I removed my eye from the eyepiece of the zoom when it was attached to the magnifying lens while holding the funnel away from the eyepiece and straight in front of me , in other words when I would expect to see a blur, I could see a clear image of what was beyond the microscope on the edge of the eyepiece lens , as though the objects in front of us were first being reflected onto our own eye before being reflected onto the zoom. Although there might be other explanations, this would be consistent with what I observed in the science section of the Newark Museum, New Jersey, when there seemed no other mechanism by which the objects beyond the box could be observed on the screen on the nearside of it. On the other hand, I have not been able to get the same result during a recent observation with the Sunagor and so will need to repeat the observation under different conditions.
Since then I have estimated approximately 14 times using a tape measure (7mm visible on a screen estimated to be about 100 mm), which is closer to what I had originally thought, which was that the microscope magnified by about 12 times (rather than the 196 stated, unless of course magnification is thought of as area). This might indicate I have underestimated the width of the film when using the Apex Learner and so I will need to repeat the observations but also with another object, which of course I need to do anyway to support the claim that the image is a constructed one.
The first observation with the Apex Learner was done using a piece of bronze film – the ocular lens wrapping - cut to approximately 1 mm wide and assuming a screen diameter of about 60 mm with 10x ocular lenses and 80mm with 20x – the actual width is difficult to gauge and the inclination is to underestimate it so as to confirm the hypothesis of low magnification, but the assumptions are based on comparing the circle one sees and any line across it, such as the lash of the eye, with circles of different diameters and a tape measure Lighting was from below and above; the circle tends to appear larger if we have been looking at measured circles and then at the screen than if we take a break and then go to the screen before looking again at the circles. Subsequent observations were made : using a tape measure, assuming marks of about ¼ of a millimetre wide between the millimetres or a red thread of a similar width (ie, ¼ of a millimetre), and a page of writing, in both cases using lighting from above. Screens of 60 and 80 would represent about 6-12 and 8-16 x magnification of the objective lens tube depending upon where in the tube the lens is and assuming we are viewing the lens tube rather than the condenser or cylinder. The observations were done forgetting I could adjust the position of the slide, although this demonstrated the precision necessary even at fairly limited magnification, and allowed me to see magnification using the 20 x and 40 x, which I was unable to focus on when the film was under a slide casing and the objective lens lowered.
(1) With 10x ocular and 4x objective – ie, a stated magnification of 40 x – the piece of foil appeared to take up about 1/10 to1/4 of the screen or about magnification of 6 to 30 x, the difference appearing to do with the amount of light distortion; however it is positioned, however, the image is not blurred and not at all complex - ie, a wash of bronze; we can see a clear image if we lift the foil from the slide closer towards the aperture; then we can see a remarkably complex image of light and dark taking up 1/5 to 1/6 of the of the screen, a magnification of about 10 to 12 times.
(2) With 20 x ocular and 4 x objective – ie, a stated magnification of 80 times – the piece of foil appears to take up 1/3 to ½ of the screen but is not clear or complex; if we lift the foil, higher than with the 10 x ocular, we again see a complex image, this time taking up ¼ to 1/3 of the diameter of the screen , but clearer at ¼, or a magnification of about 20 x.
(3) With 10x ocular and 10 x objective – ie, a stated magnification of 100 x – the foil takes up ½ to a whole of the screen – ie, a magnification of 30 x to 60x, the difference appearing to be light distortion, and the foil appearing as a very pale bronze, the illumination apparently compensating for greater opacity (as with the 10x and 20x lenses in i and ii above); if we hold the foil half way between the slide and the plane aperture, we see the thread taking up about 2/3 the width of the screen, an apparent magnification of 40 x of a remarkably complex image, with a high degree of clarity, although some blurring and colouring at the edges.
(4) With 20x ocular and 10x objective – ie, a stated magnification of 200 x – the foil is now a darker shade of bronze, implying greater opacity, and appears to take up the whole of the screen; with the 10x objective, less of the screen appears to be visible at one time than with the 4x, as we would expect with greater convexity; the image is unclear, no more than an undifferentiated wash, although with the 20 ocular, the cornea of our own eye is more visible than with the 10x and we can see the beginning of a fixed structure, mainly a few dark spots; as one lifts the foil, it again comes into focus and covering a similar width of the screen, although more obvious than with the 10x ocular is that it is difficult to view the whole of the image in focus, although this might be to do with a slight folding in the foil, but also that it comes more into focus as we lift our eyes from the eyepiece, so that the apparent magnification decreases, the size of the screen appearing to be smaller; it therefore may be unlikely that we are obtaining more than 40x magnification.
(5) It is difficult to obtain consistent results at 4, 10, 10, and 20 and the observations need to be repeated.
(6) At 10x and 40x – ie, a stated magnification of 400 x – a complex image is revealed on the screen whether or not anything is present; the corneas of the eye are present above it; and the foil appears as a pale film over the image; I was able to observe the structure of the foil when I lifted it this time the screen appearing about 50 to 60 mm and the foil width being about double that, which would imply a magnification of around 100 x; it is still complex and reasonably clear, although judgement is perhaps made more difficult by the fact that one is not looking at familiar shapes; also it is difficult to hold the foil in the same place, which in itself also suggests quite high magnification.
(7) At 20 and 40 x – ie, a stated magnification of 800 x – I could still find a relatively clear complex image of the foil when lifted it from the slide and placed quite close to the lens, with my eye still quite close to the eyepiece, but with not significantly greater enlargement than with the 10x – ie, the width of the foil did not have to travel across 10 to 20 screens before it was no longer visible; the image was darker, and fairly difficult to focus on, especially since the fixed image and corneas were also present, and the individual parts seemed larger rather than that the object was more complex than at lower magnifications, although this needs to be established with more observations; but it was still fairly clear, at what looked like a magnification of at least 100.
Further observations to those above and those following the introduction will be done with objects where it is easier to establish the extent to which clarity decreases with higher magnifications than with those that will necessary resemble the structure of living tissue more closely than that of the foil, although it also needs to be established whether more complex structures than those observed in the foil would be revealed further away from the lens with different objects, which seems unlikely. The magnifications estimated were based on measurement of length. If magnification is taken to be measurement of area, then I have in fact underestimated apparent magnification, which would be greater than 800 times.
Further observations:
(1) With 10x ocular and 4x objective lenses, magnification appears to be about 40 x using a piece of tape or a thread because the line or thread is visible on about one third of the screen; however the line is very blurred and paler than the original, nor is any ‘structure’ revealed.
(2) However, if I place a piece of translucent bronze foil about 1 mm thick under the lens, it seems to take up about half the screen, implying nearer to 20x magnification, so that some of the magnification one saw of the red line might have been distortion.
(3) Lit from below, it seems to take up less of the screen, one third or less (if one tries to see the screen at its widest, ie, with one’s eye to the eyepiece, the light is very bright), but with less distortion, ie, a clearer outline, and implying magnification of around 13 times.
(4) With 10x and 10x lenses, and lit from above, the thread appears to cover the screen but not much more of it, implying a magnification of near to 100 but with considerable blurring and with possible overestimation of the width of the screen in the cylinder; however, the structure of the thread becomes clearer at lower magnification closer to the aperture, ie, with small threads at an angle from the main thread.
Additional points: With 4x, 10x and 40x objective lens tubes alone – ie, with the eyepiece removed and when peering down the cylinder - we appear to get disproportionate magnification, with about 20mm of the tape being visible with the 40x and only 3 mm with the 4 x and 4 mm with the 10 x. This would seem to be, first, because the light above the lens illuminates a larger area than that of the circle underneath the opaque tape. The fact that one appears then to see a larger area with the 40 x may be that the aperture in fact acts as a condenser whereas there is more diffuse illumination onto the larger apertures so that we have only partial reflection onto the lenses. On the other hand, there may be some interplay between the lenses, implied also by the 4x, 10x and 40x, but further thought and observation is needed. Although 4x, 10x, and 40x lenses might have been chosen to suggest compounding, in whichever direction, of magnification within the objective lens system, I have not yet thought through how this might be or not be achieved. The 20x ocular lens is shorter than the 10x and has a smaller lens. With the 10x ocular lens alone, ie, attempting to use it with a magnifying glass, there is magnification probably closer to 5x than 10x, but the object is blurred and upside down. With the 20x ocular lens, there is relatively clear low magnification if we have our eye away from the lens but as we attempt to increase magnification by moving our eye away further there is blurring and we see the image move upside down. It does look as though one might see the lens of one’s own eye in an upper layer since the image appears to change with different eyes although that might be that I am much more short sighted in one eye than the other. One appears to be viewing something projected beyond the ocular lens because I cannot see the pink ‘wash’ from the slide with the left eye. With lighting from below only I can see the pink wash of the stain on the slide with some difference in shade of pink with the different objective lens tube magnifications consistent with lower and higher magnifications. With the addition of a second lens, we might therefore be expected to still distinguish areas of white and pink with the lower magnification lenses but an undifferentiated lighter pink with the higher magnification where we have many lines close together, which is somewhat consistent with the point above, although better conclusions could be drawn with more observations or perhaps different eyepiece lenses.
Second observations with the Apex Learner:
These were with the 10x eyepiece lens only and using the same piece of foil as the previous month, the width of which is difficult to measure with accuracy because it is not of exactly the same width, is folded in the middle, and perception of whether the semi translucent foil covers one or two millimetres can be difficult to determine. However, in basing on what follows on the assumption that the foil is only 1 millimetre wide, I will not be underestimating magnification because it can seem as much as 2 mm in places. This time the piece of foil was on the slide and with a slide casing fastened on top, although it would have been easier to manipulate had it been placed horizontally rather than vertically, which I will do for the next observation with a more carefully cut, if I can, piece of foil. I did not measure, because of the manipulation problem, the amount of the stage visible.
(1) With 10x eyepiece and 4 x objective lens: the screen this time appeared to be 100 mm, although as last time, appeared of a smaller diameter when I went back to it. The width of the foil seemed to be about 30 mm, by comparing it with the length on the tape measure rather than relative to the screen. An illumination of 30 is not much lower than the 40 stated.
(2) With the 10x and 10x eyepiece and objective lenses, the screen this time looked somewhat smaller and then 100 mm again and this time it was easier to estimate magnification by comparing it to the diameter of the screen: the width of the ribbon appear to be about the width of the screen, which would be consistent with a 100 mm length diameter. Also, there was better resolution, in the sense that it was easier to distinguish between parts in an object within the ribbon, although they could not yet be seen clearly. By easier to see, I mean that I had more of an impression of lots of parts in a small flower-like object but it is easier to distinguish and count with the 10 x objective.
(3) With the 10x and 40x eyepiece and objective lenses, I judged that the ribbon covered about 2.5 times the width of the screen, which again I estimated to be about 100 mm, which would mean a magnification of 250 rather than 400,. Given the greater discrepancy here, I will repeat the observation with a ribbon placed horizontally. However, of interest was that the image was clear and small parts were easier to see, again in the sense of attempting to count the number. While the smallest parts were discernible but not entirely clear there was a clearer outline of the flower-like object than with the 10x eyepiece and so in this sense there is better resolution with the higher magnification. However, the extent of the magnification will need to be determined with another observation.
Further observations to those above and those following the introduction will be done with objects where it is easier to establish the extent to which clarity decreases with higher magnifications than with those that will necessary resemble the structure of living tissue more closely than that of the foil, although it also needs to be established whether more complex structures than those observed in the foil would be revealed further away from the lens with different objects, which seems unlikely.
Appendix 2: the diameter of the aperture on the Prinz 2801
In theory, a convex lens – eg, one resembling a glass bead in shape - might enlarge an infinitesimally small speck to the width of the aperture or lens; whatever the difficulties and consequent distortion and weakening of the image, the size of the apertures are not correct, even allowing for approximation of measurement. The following measurements are approximate, it being difficult to measure exactly because of the obstruction of the microscope stage. They were also based on the assumption that the aperture would be too small rather than too large and so the calculations were carried out at the lowest point possible for the lens to be.
Magnification from the objective lenses of the |Prinz 2801 is said to be 60 x, 30 x and 10 x.
Actual aperture diameters: 3 mm (60 x); 1 mm (30 x); 2 mm (10 x) : for the same length of objective lens tube we would expect a larger diameter with a lower magnification because the angle of reflection is smaller, assuming the diameter of the lens is the same; in fact, the aperture for 30 x is smaller, not larger; the aperture for 10 x is larger than for 30 x but the tube is shorter and the aperture size is smaller than for 60 x.
Below I have calculated the size the aperture should be assuming: light from a convex lens of the same widths and in different positions in the microscope (i) to (x) and a ratio of reflection of 60, 30 and 10 times from slide to an 8 mm diameter lens in the objective lens tube (xi to xiii); assumed sizes based on measurement rather than calculation. Since the initial calculations are based on simple ratios only, the last three only need be read.
(i) assuming lens 8 mm diameter, 23.5 mm from slide casing, aperture 5.5 mm from lens (60 x and 30x): aperture size: (5.5 divided by 23.5) x 8 = 1.87 mm (to 2 dp);
(ii) assuming lens 8 mm diameter, 23.5 mm from slide casing, aperture 11.5 mm from slide casing (10x): aperture size: (11.5 divided by 23.5) x 8 = 3.91 mm (to 2 dp);
(iii) assuming lens 8 mm diameter, 13.5 mm from slide casing, aperture 5.5 mm from slide casing (60x and 30 x): aperture size: (5.5 divided by 13.5) x 8 = 3.24 (to 2 dp);
(iv) assuming lens 8 mm diameter, 13.5 mm from slide casing, aperture 11.5 mm from slide casing (10 x): aperture size: (11.5 divided by 13.5) x 8 = 6.81 (to 2 dp);
(v) assuming lens 20 mm diameter at top of lens tube and at height of condenser; 23.5 mm f rom slide casing; aperture 5.5 mm from slide casing (60x and 30 x): aperture size: (5.5 divided by 23.5) x 20 = 4.68 mm (to 2 dp);
(vi) assuming lens 20 mm diameter at top of lens tube and at height of condenser; 23.5 mm from slide casing; aperture 11.5 mm from slide casing (60x and 30x): aperture size: (11.5 divided by 23.5) x 20 = 9.79 mm (to 2 dp);
(vii) assuming lens 50 mm diameter, ie width of condenser (presenting problems of viewing directly and reflection if lit by mirror); 27.5 mm from slide casing; aperture 5.5 mm from slide casing (60x and 30 x); aperture size: (5.5 divided by 27.5) x 50 = 10;
(viii) assuming lens 50 mm diameter, ie width of condenser ; 27.5 mm from slide casing ; aperture 11.5 mm from slide casing (10 x); aperture size: (11.5 divided by 27.5) x 50 = 20.91 mm (to 2 dp);
(ix) assuming lens 30 mm diameter, ie width of lens tube (assuming direct viewing of screen); 53.5 mm from slide casing; aperture 5.5 mm from slide casing (60 x and 30 x); aperture size: (5.5 divided by 53.5) x 30 = 3.08 mm (to 2 dp);
(x) assuming lens 30 mm diameter, ie width of lens tube; 53.5 mm from slide casing; aperture 11.5 mm from slide casing (10 x); aperture size: (11.5 divided by 53.5) x 30 = 6.45 mm (to 2 dp);
(xi) assuming magnification from 0.133 mm to 8 mm lens at height of condenser (60 x): lens at 23.5 from slide casing; aperture at 5.5 from slide casing: aperture size: 0.133 x (5.5 divided by 23.5) x 8 = 0.25 mm (to 2 dp);
(xii) assuming magnification from 0.27 mm to 8 mm lens at height of condenser (30 x) : lens at 23.5 from slide casing; aperture at 5.5 from slide casing: aperture size: 0.27 x (5.5 divided by 23.5) x 8 = 0.51 mm (to 2 dp);
(xiii) assuming magnification from 0.8 mm to 8 mm lens at height of condenser (10 x): lens at 23.5 mm from slide casing; aperture at 11.5 from slide casing: 0.8 x (11.5 divided by 23.5) x 8 = 3.91 mm (to 2 dp).
If the aperture should be either closer to the lens or larger, as is the case with the higher magnifications, this suggests one of two things: (i) we would not see the object because it is beyond the focal point of the lens; (ii) the image would be reflected onto a part of the lens only so that, given the constriction of the lens tube and cylinder, the maximum possible enlargement would not be obtained. Crowding of light around the entrance of the aperture would also undermine the function of the condenser, which is to concentrate the flow of light.I
If it should be either further from the lens or smaller, as is the case with 10x, then this would also not be optimal because the light would not be guided sufficiently, resulting in a less clear image.
Appendix 3: Cancer
Cancer, or what is said to be the proliferation of malignant or invasive ‘cells’, seems likely to be a fictitious disease that was invented for political reasons. There is not an adequate account in the medical literature of what causes a cell to become harmful, nor a clear definition of malignancy, even if it were true that one could photograph inside the human body or view cells under a microscope, nor an adequate account of what it means for a cell or tumour to invade surrounding tissue or travel to distant organs, nor of how one cell would be able to alter another or otherwise cause harm.
It is not clear how a cell would alter so that its behaviour became destructive to other cells or the mechanism by which it would be able to cause harm. If the mechanism Is mutation, whether or not, or how, this occurs, it seems implausible that a cell would change, either as a result of loss of its normal control mechanisms or by design, such that it would proliferate and set out to destroy its host. A knock or other injury, for instance, might lead to a growth of tissue around the site of injury. But that the proliferation of cells would be caused or accompanied by an alteration in its genetic material, such that it no longer supported the body but set out to harm or kill it seems contrary to what we know about the behaviour of living things.
First, the medical literature is not consistent as to whether cancer is diagnosed as a result of cell pathology or invasion of local tissue. In terms of cell pathology, it is not clear how ‘cells’ can be viewed, since microscopes enlarge rather than see below to any underlying structure (where the various parts of the cell are said to lie, whether or not the cell is opaque). Even if the magnifications claimed were possible to achieve (ie, in terms of lenses), the images one would see at such magnifications, from observation of camera lenses, would be too blurred to identify something the size of a cell. From observation, the size of the image one sees most clearly under a microscope is the same as that of the object itself viewed at close range, such that any magnification blurs to some extent. This is consistent with what happens when one magnifies using a camera or with the blurring of larger letters at the periphery of one’s vision that can occur when one is reading.
The image of a cancer cell could not be seen, to use Descartes’ phrase, clearly and distinctly. Instead, the microscope acts similarly to a kaleidoscope. First, a microscope would not be able to view objects the size of cancer, viral or Ebola cells because the image would blur at the magnification needed, as one can see from using a camera, which can bring things closer but not magnify without losing clarity, so that what one cannot see with the eye alone is not likely to exist. Second, when someone looks into a microscope they are not focusing on the glass slide, but on something positioned before it within the cylinder: their focal length is shorter than the distance between their eyes and the slide. This can be tested by removing one’s eye from the eyepiece and noticing that one has to adjust one’s vision before being able to see a similarly small object placed on, or by, the slide. Whether the adjustment is the result of the difference in lighting or the length of the cylinder or position of the object, one is not focusing on the object on the slide. Whether or not the object on the slide is magnified, or by how much, when one looks into the microscope one is viewing very small objects as if they were not magnified, ie, the objects may alter in size when magnified but one’s vision does not. In other words, one is looking at magnified objects as though one were looking at larger objects with the eye alone. Therefore, by looking into the microscope cylinder one adjusts one’s focal length so that the object on the slide would actually blur, or appear smaller, because beyond one’s normal focal point, than if one were viewing it with the eye alone, ie, without a microscope. Third, even if one could see the objects on the slide – ie, if one’s view were not obviously focusing on something within the microscope – they would appear smaller than one viewed by the eye alone because the effect of looking at something from a distance through glass is to reduce, rather than increase, its size, the more so the greater the thickness of the glass, I assume again because of the effect of the glass barrier on focal length.
Although it might seem possible that an image of the material on the slide is projected onto a screen within the microscope, rather than viewed directly, the arrangement of the microscope makes this unlikely even if the objects one views looked like they might be projected, and some of them do not. The arrangement of the microscope does not make projection seem all that likely: one would expect the slide to be encased, so that there is no possibility of diffusion before light also has to enter the object lenses, before magnification within the microscope takes place, and in such a way that the material to be viewed is not obstructed before it is magnified, by the glass on the microscope on the stage and on the slide itself. In fact, if I remove the glass slide using my own microscope the image does not apparently change. Also, while the more fixed objects at the lower end of the cylinder have a shadowy look that might suggest projection, this is also an effect of magnification, ie, of blurring, while the objects nearer the eye have a more translucent look.
My own microscope, a Prinz Opti-Craft Microscope Outfit, Zoom 100x to 900x, purchased in the late 1960s, contains a slide with a butterfly scale on it contained within a glass cover slip. With a concentrated beam of light, it can appear as though the light captures the red scale such that a red ‘wash’ appears in the cylinder, although at other times when the image on the screen is present, there is no colouration of the image. Whether or not the mark is too small for it to be seen if the slide is removed or present, there is no significant magnification if its presence cannot be detected. On the slide there is an opaque, cream-coloured, label, marked ‘Butterfly Scale, Japan’ (where the microscope was made). If one turns the slide over, there is a similar, but smaller, mark, ie, apparently a second scale, or part of one (the first is rectangular, the second circular, so together they might resemble the marks of a code), in the glass of the slide behind the label. Although I had originally thought I had detected this scale when using the microscope with sufficient light penetrating the label to illuminate the screen, thinking that the point being made might be that the light from the mirror could illuminate material in the first slide but not the cover slip (but still the cylinder), I have not seen it subsequently. It seems much more likely that the point being made is that just as the second scale had been hidden by the semi opaque label, so any material on the slide is hidden by a semi opaque object within the microscope. The microscope apparently has a magnification of 100 to 900 times, although only the focus is labelled lox and lox. However, if there were more powerful magnification lenses within the microscope, would they reach as far as the material on the slide even if not blocked by an opaque material of some sort? Similarly, if the microscope were internally lit, one would expect the focus of the light to be the object on the slide., but there is no beam of light with a traditional microscope. Also, from observation, if the light, whether from behind or from the mirror, is too great, the image in the cylinder will blur, in the same way that one sometimes only sees a blur when looking into a camera in bright light. Finally, whether or not one squints when looking into the cylinder one’s focal length is less than that needed to see clearly the objects on the slide, even if one is not apparently squinting, as can be observed if one removes one’s eye from the eyepiece. On the other hand, if there were such a powerful zoom lens within the microscope (100 x to 900 x), ie, beyond the effect created by the cylinder itself, one would in fact expect the object to be further away, since the effect of a zoom, as with any magnification, is to extend one’s focal length. Although the fact that and the reasons why one is not able to be able to see what is not on the slide need to be re-tested in different lights and to be better thought through, once one doubts that one is viewing what is on the slide it seems almost certain that one is viewing something contained within the microscope as well as, in some lights, the lenses of one’s own eye projected above it.
Instead, when someone looks into a traditional microscope, upon which the science of diagnosed disease is based, they are not viewing the material on the glass slide but an image within the cylinder. If one illuminates the cylinder of the microscope with a mirror one sees the same image as when the microscope is lit with batteries or an electric current (which were an evolution from the original way of lighting the microscope with a mirror). When using the mirror to light the microscope one may see little or nothing in the way of an image on the screen of the microscope, even if there is enough light to illuminate the cylinder and screen (ie, it appears blank) but if brighter natural or artificial light (but not too much) is captured by the mirror, an image appears on the screen and before it (ie, closer to the eye). The upper image has a more layered and translucent look and appears less fixed than the image on the screen.
Looking into the microscope, one can see the lenses of one’s own eye projected in the cylinder before a more fixed image on the screen. That one is seeing the lenses of one’s eye is apparent because it is an image one sees when squinting into the light. Looking in the microscope the effect is achieved whether or not one squints, if one closes the other eye, because of the relative darkness of the cylinder. The more fixed image on the screen has a more shadowy look than the brighter, translucent, image of the lens of one’s eye, suggesting it might be projected from below, rather than illuminated by, the screen, or the shadowy look might be the result of greater magnification – ie, it represents a loss of clarity. It also has a denser, more detailed, look (even though the projected image of the lens of one’s own eye becomes denser as one moves one’s, concave, eye away from the eyepiece and looks into it, such that the image appears smaller, as more compact, than if one were not looking into the glass).
Although there are a greater number of objects within the image on the screen than in the image of the lens of one’s eye (but nor do they take on the ‘pixel’ effect one sometimes see when, for instance, opening one’s eyes in the morning after sleep, resembling something more like a road map), the basic structure, when using my own microscope, is not that different nor the resembling objects of the structure of a different size, from the image of the projected lens of one’s own eye. For example, when viewing the projected lens of one’s own eye, one sees translucent ‘ribbons’. The image on the screen has ribbons of a similar length and width, albeit with a darker and more shadowy look and with ‘knots’ in them. Although the more shadowy look suggests a greater magnification, this might be the result of projection and the greater number of objects because they are better illuminated. Although many things in nature mimic other things, the similarity of the structure lends some support to the idea that one is viewing on the screen an object with similar characteristics to the lenses of one’s own eye and at a similar magnification, which is not significantly greater than that done by the eye itself when squinting into sunlight.
Examination of the ‘objective lenses’ at the bottom of the microscope suggest that what one may be seeing when looking into the microscope is, in fact, the lens of a smaller eye, since, without wishing to take my own microscope apart, I can see what resembles the hard orange ‘iris’ of a bird (it particularly looks like that on a pigeon) inside the larger of the three objective lenses of the microscope, and on two when photographed and magnified. When moving the largest of the three objective lens, the iris type object becomes more or less visible, as though the round object has been placed between two lenses, or bits of glass, but occupying a slightly smaller space.
An image of this object may be projected directly by the light from the mirror onto the screen, although if one tilts one’s eye one can see smaller images to the left and right, suggesting some sort arrangement of mirrors, as in a kaleidoscope. The magnification is probably comparable to that achieved by the eye and the eyepiece, since although the image is more crowded than that of one’s own eye, the width of the ‘ribbons’ is similar, suggesting that the difference between the two materials is a result in their different characteristics and better lighting rather than a difference in magnification. This is plausible also if one thinks that one is not seeing the whole of the visible lens of one’s own eye when squinting into the eyepiece, ie, the image of the lens of the smaller eye could fill the screen with a similar magnification if placed in the appropriate position.
In any case, setting aside the fact that one is unlikely to be viewing biopsy cells under a microscope, if there appear to be regularities in terms of changes (ie, if some people’s cells, but not others, appear to change in similar ways), these need not in any case indicate life-threatening changes to cells or tissue rather than the wear and tear associated with ageing. Some terms used to denote pathology, such as ‘necrosis’, ‘stroma’ and ‘cribriform’ and even ‘calcification’, are difficult to pin down in terms of why they are dangerous or by what mechanism. For example, ‘necrosis’, associated with malignancy, refers to exaggerated cell death (as opposed to ‘apoptosis’, which is normal cell death) and is an indicator of uncontrolled proliferation, apparently because in an area where you have more cells, or tissue, you would be more likely to have increased cell or tissue death. But increased cell death would suggest either a check on unnatural proliferation or would, in any case, also be associated with benign tumours or, if not, suggest that benign tumours are more likely to be invasive, in the sense of growing sufficiently to invade surrounding tissue, than malignant ones.
If cancer is not diagnosed according to particular features of the cell (nor by cell growth since this is also a feature of benign tumours) but by invasion of surrounding tissue, this would seem to imply producing changes in surrounding cells or tissue, since otherwise a benign tumour could also potentially obstruct surrounding tissue. It is not clear by what mechanism one cell would invade another or what this would look like in cell cytology/histology. If surrounding tissue also changes, other than because of force or obstruction, it is likely to be as a result of the same factor(s), including any viral agent, that caused the initial cell to change, rather than that the initial cell invaded the other, unless the process being described is reproduction, or replication, involving, as is said to be the case with viral cells, some sort of interaction between cancerous and non-cancerous cells.
The presence (or spread) of tumours is usually detected, or confirmed in the case of those that can be felt by the hand, by x-rays or CT scans. Again, no camera, whether or not it is said to emit ‘radiation’, can see beneath the surface of the human body, including in order to see a cell or ‘gland’ that has travelled from another part of the body. The principle by which an MRI scan works seems more plausible, ie, that it produces images that reflect the attractions of different substances within the body to a magnet, but in practice it would not be sufficiently precise, given that the body has depth as well as area.
If invasion is by the tumour itself, rather than by a single cell, setting aside by what process the tumour itself formed (ie, whether through invasion of one cell by another, including in order to replicate, in which case it would be difficult to distinguish between the formation of the tumour itself and invasion of adjacent tissue, or through a spontaneous proliferation of malignant cells), again, does it occupy the adjacent tissue or cause it to change? If the tumour only moves, or displaces, adjacent tissue, this too would also be a feature of benign tumours.
Second, the mechanism by which a cancer cell alters, harms or kills a host cell and ultimately its host seems to be asserted rather than explained, which would be consistent with the ambiguity as to whether cancer is diagnosed by malignancy or invasion of surrounding tissue. If the mechanism is force, an obstructive tumour is as likely to be benign as malignant. If the mechanism is individual cell mutation, it is not clear why cell division would take place within the body, unless the body grows to adulthood in a way approximating cell growth, although the splitting of cells into identical ‘daughter’ cells would not in itself appear to account for either growth or differentiation within the body. But neither is genetic mutation explained satisfactorily.
If environmental factors (or ageing) cause an alteration in RNA/DNA then it is not clear why these factors are not themselves the cause of ill health in that there appears to be no satisfactory account or description of the means by which alteration in information stored in the cells causes an alteration in bodily tissue. For instance, there is understanding of the link between inputting information into a calculator or adding computer software and the changes that appear on the screen. There is a plausible explanation of the changes to the body when a surgeon follows a particular set of instructions. In each case, there is an understanding of, first, the agency, in the sense of there being a person operating the calculator or computer or on the body, second, of the mechanical link, in the sense of what follows when one presses keys on the calculator or inserts software or makes an incision, and, third, of the force needed, either electrical or human. There is not a comparable explanation of the agency and mechanism of change within RNA/DNA. RNA/DNA, or the genetic information/instructions it contains, is said to have an independent existence and be capable of agency in the sense that it is present in the body and influences it either in the absence of environmental factors or because it alters the character of environmental consequences (since otherwise it would be unnecessary in the explanation of changes to the body). But if it does have agency, there is no adequate account of how a genetic code, an abstract entity, would have the capacity to produce changes in the body or through what mechanism (ie, what type of mechanical link). Nor can there be if, first, it is not possible to view the body clearly at the scale necessary to view changes and, second, if there is no satisfactory philosophical explanation of the link between, or ontological compatibility of, genetic information and bodily tissue.
Third, metastasis is said to be the process whereby a cancer that starts in one part of the body kills, by spreading to distant, vital organs. A cell from the primary tumour is said to break loose when it loses its ability to stick to the initial tumour and then travels along the blood stream or via the lymph system. In the distant organ, the cell would regain its ability to stick and then begin to proliferate. The new mass of cells would be referred to as a secondary tumour – eg, a thyroid tumour in the lung, composed of thyroid cells rather than lung cells. That different parts of the body begin to develop tumours spontaneously, or even that one part of the body would copy another, would seem more plausible than that there are secondary tumours consisting of cells that have travelled from the initial tumour.
How would they travel? An apparently infinitesimally small ‘cell’ would be unlikely to be able to travel within the relatively thick blood or ‘lymph’, especially from a lower part of the body to a higher, or even if it were able to travel in any spaces between blood or lymph cells and upwards, be able then to enter distant organs, ie, penetrate, for example, the membranes of the brain or lungs. (And why never the heart?) From observation, larger, not smaller, animals have less difficulty surviving for any length of time in water, while smaller fish will have more difficulty with relatively viscous fluids, such as blood.
CT scans apparently show single cells that have travelled to another organ but it seems unlikely according to common sense (which is an abstraction from observation of comparable sensory perceptions) that a scan would really be able to distinguish a single cell even if it were possible to photograph what is inside the body. If, instead, a large number of cells broke away, what, setting aside their motive, would propel them to a distant organ? The hypothetical force exerted by any number of cells that remain invisible to the eye would still seem likely to be insufficient even if each contained a motor of some sort, which they are not said to do. Whatever the stated facts of the blood stream, it also seems unlikely that this alone would be able to carry cells, particularly from a lower part of the body to a higher.
Fourth, what is usually said to kill in the case of cancer is a recurrence, because a recurring cancer is said to be more aggressive, which one assumes means it would either grow more quickly or show greater pathology and propensity to metastasise, although in either case it seems uncertain by what mechanism a cancer cell harms and ultimately kills. If the cells, or tissue, are malignant, and become more malignant as they ‘mutate’, it is not clear in what sense this could be true - other than that they consisted of parts of cells that were said to indicate malignancy - if they do not themselves contain agents of harm or are not able to otherwise cause alteration to cells or tissue.
If recurrence and mutation mean they are able to proliferate more quickly, aside from the fact that this need not indicate malignancy it is not clear, why and how this could be true. If one assumes agency of some sort, then why, after surviving an attempt to eradicate it, would it seek to destroy its host (and therefore itself) or otherwise draw attention to itself? And if recurring cancers are more dangerous than the initial cancer, and often fatally so, then why do oncologists treat the less aggressive, indolent, cancers instead of monitoring them, when surgery and anaesthesia, and, apparently, ‘radiation’, are themselves dangerous. It also seems unlikely that drugs would be able to target parts of the body as precisely as we are told they can, or that they would not all have some impact on both the stomach and the head.
Fifth, the benign growths, or lumps of altered, tissue, caused by such things as knocks, burns, and sun exposure, might cause obstruction if they become too large, but in most cases they do not seem to grow, so that more damage is likely to be done by attempting to remove them surgically than by leaving them. They may even have grown, after an injury, to protect a part of the body from further damage. The most likely causes of the growth are the hardening of tissue and the accumulation of fluids after injury. However, it seems unlikely that tumours would grow and expand to a considerable size within the body, especially as we age and weaken, unless, again, their purpose is protective. In any case, those growths we cannot see or feel, we have no certain means of knowing whether or not they exist. Therefore symptoms such as a persistent cough are likely to indicate weakness or irritation rather than disease. (In the same way, a cold is a sign that one is weak. You do not catch a cold, which is the body’s way of warming up or expelling liquid). Similarly, a shadow on an x ray is only likely to be a lighter area on a photographic negative (unless a stock image is produced).
What kills in the case of apparently fatal illnesses such as those associated with cancer (and other diseases) is a cumulative weakening that may be partially caused by medication (prescription or otherwise), especially when there are initial unpleasant side effects, but is more likely to be caused by other factors, physical and psychological, in the environment of someone diagnosed with a viral infection (or other disease). These include such things as alcohol, tobacco, and non-prescription medications and well as narcotics. Other factors include surgery, which weakens, at least temporarily, so increasing the risk from other depressants, because of anaesthesia or blood loss; poor diet (bad or insufficient food); environmental factors, particularly inadequate heating, extreme heat, fluctuations in temperature, utility emissions; excessive physical exercise or overwork, or too little exercise; insomnia (eg, caused by stress or insufficient food), or too much sleep (which may cause headaches). Perhaps decisive, for example, with respect to appetite, is the psychological stress and fatalism caused by the belief that one has a potentially fatal illness.
It would strike most people as inconceivable that diseases such as cancer could be invented fictions, and so we have not thought about whether they are plausible according to observation, common sense or logic. However, the term, the Final Solution (associated with the Wannsee Conference, which historians now say may have taken place in 1942, the year of the ‘Beveridge’ Report, which envisaged a health service that would deal with the five giants of disease, poverty, ignorance, squalor and idleness), might have implied the use of intravenous fluids and alcohol in order to reduce and control populations.
Subscribe to:
Posts (Atom)